Survival Strategies Used by Salmonella to Persist in Dry and Low-Moisture Processing Environments
ID
FST-487NP
Introduction
Salmonella contamination in dry and low-moisture food processing environments is a food safety concern recognized by the FDA, with the organism being responsible for an estimated 94% of recalls of low water activity foods in the United States (Santillana Farakos and Frank 2014). Salmonella makes its way into dry and low-moisture processing environments in ways that are similar to what is seen in higher moisture processing environments, including via raw materials (e.g., packaging materials, ingredients), air (e.g., air handling systems, receiving areas), water (e.g., sanitation), equipment or tools, employees or visitors, and pests (Beuchat et al. 2011), as shown in Figure 1.
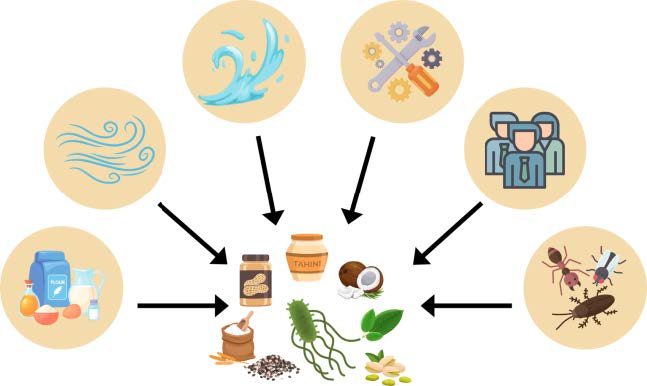
For a microorganism to grow in a food processing environment, there must be enough available moisture for the organism to use. In a dry or low- moisture processing environment, there are typically not enough consistent sources of moisture for microorganisms to grow; however, Salmonella has shown the ability to adapt to this low-moisture stress and survive for long periods of time, sometimes gaining the ability to withstand other stressors as well (termed “cross-tolerance”) (Finn et al. 2013).
Adaptive Behaviors Used by Salmonella
Salmonella has been shown to use several behaviors to adapt to stressors and survive in dry or low- moisture environments, including accumulation of osmoprotectants, up-regulation of outer membrane porins, alteration of gene expression, rRNA degradation, entering a viable but not culturable state, filamentation, and biofilm formation (Finn et al. 2013).
Osmoprotectants
When Salmonella is exposed to a low water activity environment, the organism needs to balance the osmolarity of its internal cell composition with that of the external environment (Malakar et al. 2022). In a dry or low-moisture environment, the solute concentration on the outside of the bacterial cell is higher than inside (termed “hypertonic”), often resulting in extreme water loss and shriveling of the organism (Figure 2).

One of the ways Salmonella prevents this water loss is by using osmoprotectants (Finn et al. 2013, Li et al. 2012). Osmoprotectants are small, neutral molecules that are non-toxic at high concentrations. Salmonella can increase the internal amount of osmoprotectants by either synthesizing them internally or by transporting them from the environment into the cell. Salmonella can do this in as little as six minutes after the introduction of the stressor (Balaji et al. 2005).
Outer Membrane Porins (OMPs)
OMPs are proteins that extend across the outer membrane of the bacterial cell that help promote the passive diffusion of osmoprotectants into the cell (Figure 3).
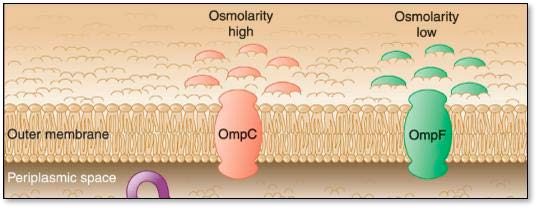
Under dry or low-moisture stress, Salmonella expresses two OMPs to bring osmoprotectants into the cell within twelve minutes of the stressor being introduced (Balaji et al. 2005, Feng et al. 2003, Wang et al. 2012).
Alternative Sigma Factors
Salmonella possesses a variety of genes that, when expressed, will result in the production of molecules (e.g., proteins) that provide advantages to the cell under certain environmental conditions or stressors. The expression of these stress response genes is dependent on the specific environment the cell is in and is regulated by alternative sigma factors. When Salmonella is stressed in a low-moisture environment, alternative sigma factors will up- regulate the expression of genes that allow Salmonella to survive (McMeechan et al. 2007).
Ribosomal RNA (rRNA) Degradation
rRNA is essential to genetic expression, and therefore the function, of a living organism (Basturea et al. 2012, Deng et al. 2012). Under stress, Salmonella has been shown to increase rRNA degradation, which is the removal of rRNA components that are not immediately functionally required under an unfavorable environment. The cell may then enter a partially dormant state, wherein it is still metabolically active but has been shown to express about 5% of its total genes to modulate its environment to prolong survival in dry or low- moisture environments (Lewis 2007, Deng et al. 2012).
Viable But Not Culturable (VBNC) State
Under certain stress conditions, Salmonella has been shown to induce a “viable but not culturable” state (Oliver 2010, Gruzdev et al. 2012, Jayeola et al. 2022). Organisms in a VBNC state are metabolically inactive while in an unfavorable environment.
Though inactive, the organism can preserve viability and regrow when resuscitated (Gupte et al. 2003), such as when reintroduced to a high-moisture environment.
Filamentation
Filamentous Salmonella cells are produced in response to environmental stress, preventing normal cellular division (Pratt et al. 2012). Formation of these filamentous cells presents a challenge in the processing environment as it adds to Salmonella’s biomass without increasing the detectable number of individual cells, which means that cell counts may underestimate how many cells are present. When Salmonella’s environment becomes favorable, these filamentous cells can rapidly divide to become viable cells that can potentially cause human illness (Mattick et al. 2000).
Biofilm Formation
Under certain conditions, Salmonella may produce biofilms to protect against excessive water loss under stress (White et al. 2006, Stocki et al. 2007). The adherence ability of Salmonella biofilms may be reduced depending on the surface (Giaouris and Nychas 2006); however, biofilm production has been shown to offer cross-protection against other environmental stressors (e.g. pH and heat).
Application to Food Processing Environments
Consideration of how a low water activity state was achieved (e.g., through thermal or non-thermal drying methods) may help predict trends in Salmonella behaviors observed in processing environments, such as increased resistance to heat or sanitizers and proficiency of biofilm formation. Incorporation of this information into hazard analyses and control plans may allow for the optimization of production practices aimed at mitigating the organism’s survival.
Additional research is necessary to better understand ways Salmonella adapts to these environments for the purpose of developing effective mitigation strategies through a combination of bench-style, applied, and intervention-specific experiments.
Research using methods to simulate food industry environments is needed to provide a better understanding of how specific Salmonella serovars behave in commodity-specific production settings (e.g., Salmonella enterica serotype Typhimurium in dried coconut, Salmonella enterica serotype Senftenberg in peanut butter). Food businesses should also work to collaborate with university and Cooperative Extension services to use experiential data collected at industry scale (such as from environmental monitoring programs or monitoring data) to predict and adapt in real-time to the changing landscape of Salmonella survival in dry and low-moisture environments.
References
Any questions or concerns related to accessing the following resources should be directed to Alexis Hamilton at ahamilton@vt.edu.
Balaji, B., O'Connor, K., Lucas, J. R., Anderson, J. M., & Csonka, L. N. (2005). Timing of induction of osmotically controlled genes in Salmonella enterica serovar Typhimurium, determined with quantitative real-time reverse transcription- PCR. Applied and environmental microbiology, 71(12), 8273-8283.
Basturea, G. N., Harris, T. K., & Deutscher, M. P. (2012). Growth of a Bacterium That Apparently Uses Arsenic Instead of Phosphorus Is a Consequence of Massive Ribosome Breakdown*♦. Journal of Biological Chemistry, 287(34), 28816-28819.
Beuchat, L., Komitopoulou, E., Betts, R., Beckers, H., Bourdichon, F., Joosten, H., ... & Ter Kuile, B. (2011). Persistence and survival of pathogens in dry foods and dry food processing environments. ILSI Europe report series, 2011, 1-48.
Deng, X., Li, Z., & Zhang, W. (2012). Transcriptome sequencing of Salmonella enterica serovar Enteritidis under desiccation and starvation stress in peanut oil. Food Microbiology, 30(1), 311-315.
Feng, X., Oropeza, R., Walthers, D., & Kenney, L. J. (2003). OmpR phosphorylation and its role in signaling and pathogenesis. ASM News-American Society for Microbiology, 69(8), 390-395.
Finn, S., Condell, O., McClure, P., Amézquita, A., & Fanning, S. (2013). Mechanisms of survival, responses and sources of Salmonella in low- moisture environments. Frontiers in microbiology, 4, 331.
Giaouris, E. D., & Nychas, G. J. E. (2006). The adherence of Salmonella Enteritidis PT4 to stainless steel: The importance of the air–liquid interface and nutrient availability. Food microbiology, 23(8), 747-752.
Gruzdev, N., Pinto, R., & Sela, S. (2012). Persistence of Salmonella enterica during dehydration and subsequent cold storage. Food Microbiology, 32(2), 415-422.
Gupte, A. R., De Rezende, C. L. E., & Joseph, S. W. (2003). Induction and resuscitation of viable but nonculturable Salmonella enterica serovar Typhimurium DT104. Applied and Environmental Microbiology, 69(11), 6669-6675.
Jayeola, V., Farber, J. M., & Kathariou, S. (2022). Induction of the viable-but-nonculturable state in Salmonella contaminating dried fruit. Applied and Environmental Microbiology, 88(2), e01733- 21.
Kenney, L. (2006). Figure presented as part of lecture entitled “Gene regulation” for the University of Washington. https://www.gs.washington.edu/academics/cours es/manoil/former/41108/lecture/lectureMarch5.pdf.
Lewis, K. (2007). Persister cells, dormancy and infectious disease. Nature Reviews Microbiology, 5(1), 48-56.
Li, H., Bhaskara, A., Megalis, C., & Tortorello, M. L. (2012). Transcriptomic analysis of Salmonella desiccation resistance. Foodborne Pathogens and Disease, 9(12), 1143-1151.
Malakar, P. K., Liu, J., Wu, Q., Zhang, Z., & Zhao, Y. (2022). Response of foodborne pathogens to osmotic stress. In Stress Responses of Foodborne Pathogens (pp. 125-140). Cham: Springer International Publishing.
Mattick, K. L., Jørgensen, F., Legan, J. D., Cole, M. B., Porter, J., Lappin-Scott, H. M., & Humphrey, T. J. (2000). Survival and filamentation of Salmonella enterica serovar Enteritidis PT4 and Salmonella enterica serovar Typhimurium DT104 at low water activity. Applied and environmental microbiology, 66(4), 1274-1279.
McMeechan, A., Roberts, M., Cogan, T. A., Jørgensen, F., Stevenson, A., Lewis, C., ... & Humphrey, T. J. (2007). Role of the alternative sigma factors σ E and σ S in survival of Salmonella enterica serovar Typhimurium during starvation, refrigeration and osmotic shock. Microbiology, 153(1), 263-269.
Oliver, J. D. (2010). Recent findings on the viable but nonculturable state in pathogenic bacteria. FEMS microbiology reviews, 34(4), 415-425.
Pratt, Z. L., Chen, B., Czuprynski, C. J., Wong, A. C., & Kaspar, C. W. (2012). Characterization of osmotically induced filaments of Salmonella enterica. Applied and environmental microbiology, 78(18), 6704-6713.
Santillana Farakos, S. M., & Frank, J. F. (2014). Challenges in the control of foodborne pathogens in low-water activity foods and spices. The microbiological safety of low water activity foods and spices, 15-34.
Stocki, S. L., Annett, C. B., Sibley, C. D., McLaws, M., Checkley, S. L., Singh, N., ... & White, A. P. (2007). Persistence of Salmonella on egg conveyor belts is dependent on the belt type but not on the rdar morphotype. Poultry Science, 86(11), 2375-2383.
Wang, L. C., Morgan, L. K., Godakumbura, P., Kenney, L. J., & Anand, G. S. (2012). The inner membrane histidine kinase EnvZ senses osmolality via helix‐coil transitions in the cytoplasm. The EMBO journal, 31(11), 2648-2659.
White, A. P., Gibson, D. L., Kim, W., Kay, W. W., & Surette, M. G. (2006). Thin aggregative fimbriae and cellulose enhance long-term survival and persistence of Salmonella. Journal of bacteriology, 188(9), 3219-3227.
Virginia Cooperative Extension materials are available for public use, reprint, or citation without further permission, provided the use includes credit to the author and to Virginia Cooperative Extension, Virginia Tech, and Virginia State University.
Virginia Cooperative Extension is a partnership of Virginia Tech, Virginia State University, the U.S. Department of Agriculture, and local governments. Its programs and employment are open to all, regardless of age, color, disability, sex (including pregnancy), gender, gender identity, gender expression, genetic information, ethnicity or national origin, political affiliation, race, religion, sexual orientation, or military status, or any other basis protected by law.
Publication Date
August 14, 2024