Nanobubbles as an Emerging Sanitation Technology
ID
FST-383NP
Introduction
The ability of bacteria to adhere to surfaces has significant implications for the food industry. Attachment of pathogenic bacteria to fresh produce and contact surfaces can increase food safety risks as these surface properties allow bacteria to securely adhere to them.
Food contact surface attached bacteria can form biofilms and these biofilms can enhance resistance to sanitation. The enhanced resistance of biofilms to sanitizers can be attributed to many factors including formation of extracellular polymeric substances (EPS) that rapidly consume sanitizers such as chlorine. In addition, repeated exposure of bacteria to sub-lethal concentrations of sanitizers in a biofilm matrix can also enhance their resistance to sanitizers. Furthermore, the lack of removal of this EPS during sanitation may result in reformation of a biofilm post sanitation, thus enhancing their persistence on food contact surfaces.
Enhanced resistance and persistence of biofilms are highly significant as approximately 65% of the foodborne outbreaks are traced back to bacterial biofilms.
Thus, the antimicrobial activity of commonly used sanitizes including chlorine, hydrogen peroxide, quaternary ammonium (QUATS), and peracetic acid can be limited against surface attached microbes due to rapid depletion in concentration and activity upon reactions with organic matters and complexity of structural features of the plant surface and biofilms.
What are Nanobubbles?
Nanobubbles are extremely small gas bubbles that due to their several unique properties, can be used for different purposes including aquaculture, hydroponics, aquaponics, removing algae bloom and microbial biofilms.
Nanobubbles production
Several different methods including cavitation, electrolysis, electrochemical cavitation, applying nano-pore membrane, hydrodynamic cavitation, mechanical agitation, sonochemistry using ultrasound, temperature difference method, alcohol- water exchange, and laser-activated nano-gold substrates have been used for generating nanobubbles. In all of these techniques, the principal behind the nanobubbles production is reduction of pressure using surface tension and energy deposit.
Nanobubbles size
Nanobubbles (NB) are less than 200 nm, which is 500 times smaller than a microbubble, and 2500 times smaller than a salt grain (Figure 1).
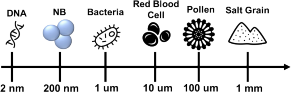
Nanobubbles properties
Surface charge: all bubbles naturally have a surface charge; however, smaller bubbles have a stronger surface charge. Nanobubbles have a negative zeta potential and charge on their surface preventing them from coalescence, and supporting their stability.
Nanobubbles are hydrophobic.
Their internal pressure is around 6 MP, which can release a high amount of energy at the nano-level.
They have Brownian motion and stay in the water column longer than regular bubbles.
- They can be filled with different gas sources.
Nanobubbles stability
Nanobubbles exhibit remarkable stability with a lifetime of hours to days. They are subjected only to Brownian motion and have negligible buoyant force resulting in high stability.
They are negatively charged at neutral pH (zeta potential below -20 mV for most gases) which the charge comes from the excess OH ions relative to H+ ions at a bubble-water interface, and the internal pressure of nearly 6 MPa. The stability of nanobubbles determines their antimicrobial properties since it has strong a correlation with surface tension and nanobubbles surface properties.
Hydrophobic materials adsorbed on the part of the nanobubbles surface can stabilize the bubble by ensuring dynamic equilibrium between mass influx and outflux.
Antimicrobial properties
Nanobubbles can penetrate into the organic materials and disrupt the biochemical structures including microbial biofilms (Figure 3).
Also, they can reduce surface tension and increase the bacterial detachment.
- Once they burst, they release a high amount of energy generating hydroxyl and oxygen radicals which can inactivate bacteria.
- Nanobubbles technology enhances microbial inactivation which combines with other technologies such as ultrasonication.
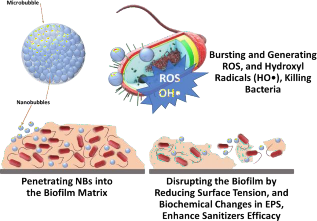
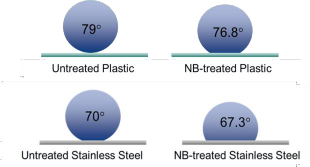
Nanobubbles Advantages
Nanobubbles can significantly remove microbial biofilms on surfaces
Nanobubbles’ efficacy will be enhanced in combination with chlorine-based sanitizers
Nanobubbles can induce microbial injury
Reduce chemical and antibiotic applications
Enhance sanitizers delivery to bacteria
Penetrate into biofilms
Proper for water treatment
Proper for agricultural water treatment
Could be used for removing biofilms from pipes
- Membrane sanitation in the food industry
Applications
Enhance plant growth by providing a high amount of accessible oxygen
Improving root structure and nutrient uptake
Increasing plants’ resistance to diseases
Improving the microbial community by promoting the growth of beneficial microbes
Improve processability of foods due to the viscosity-reducing effect of nanobubbles
Enhance the flavor of foods
Improving the health benefits of food
Nano-emulsion and drug delivery
Improving protein extraction
Improving protein functional properties
Water treatment industry
Food processing plant waste water treatment
Agricultural water sanitation
Fresh produce, and fruits washing and sanitation
Harvest and processing equipment sanitation
Pipe sanitation
Shellfish sanitation
Environmental applications
Removing toxic algae bloom
Cleaning ponds and pools
Beverage industry
Cooling towers
Wound healing
- Biomedical applications
References
Shiroodi, S., Schwarz, M.H., Nitin, N., Ovissipour, R. 2021. “Efficacy of Nanobubbles Alone or in Combination with Neutral Electrolyzed Water in Removing Escherichia coli O157: H7, Vibrio parahaemolyticus, and Listeria innocua Biofilms.” Food & Bioprocess Technology.1-1.
Rafeeq, S., Shiroodi, S., Schwarz, M.H., Nitin, N., Ovissipour R. 2020. “Inactivation of Aeromonas hydrophila and Vibrio parahaemolyticus by Curcumin-Mediated Photosensitization and Nanobubble-Ultrasonication Approaches.” Foods. (9):1306.
Additional Resources
Virginia Cooperative Extension materials are available for public use, reprint, or citation without further permission, provided the use includes credit to the author and to Virginia Cooperative Extension, Virginia Tech, and Virginia State University.
Virginia Cooperative Extension is a partnership of Virginia Tech, Virginia State University, the U.S. Department of Agriculture, and local governments. Its programs and employment are open to all, regardless of age, color, disability, sex (including pregnancy), gender, gender identity, gender expression, national origin, political affiliation, race, religion, sexual orientation, genetic information, military status, or any other basis protected by law
Publication Date
January 27, 2021