Using Groundwater for Agricultural Irrigation in Virginia
ID
BSE-215P (BSE-353P)
EXPERT REVIEWED
Virginia usually receives ample rainfall to support agriculture, but droughts in 2002, 2007, and 2010 led to severe problems for farms in many regions. Using groundwater for irrigation can help manage drought risks on farms without access to ponds or streams. However, installing groundwater wells can be expensive and time-consuming. The amount of water obtained from wells can vary in different regions of the state. This bulletin is intended to provide agricultural producers with information on technical and regulatory issues related to irrigating with groundwater. This includes:
- The factors that influence groundwater yields in different parts of Virginia.
- Permitting and reporting requirements for groundwater use in Virginia.
- The well drilling and maintenance process.
- The importance of groundwater management and sustainability.
This information should help producers evaluate whether groundwater irrigation may be appropriate for their operations. It will also help them be informed consumers if they choose to move forward with implementing a groundwater-based irrigation system.
Groundwater – A Shared Resource
Groundwater is water that lies below the earth’s surface, stored in the cracks and spaces that exist in soil, sand, and rock. Groundwater exists in two places: the unsaturated zone, where the spaces between grains of gravel, sand, silt, and soil contain both air and water, and the saturated zone, where these spaces (called pore spaces) are filled with water. While most plants obtain water from the unsaturated zone, groundwater that can be pumped to the surface must
come from the saturated zone. Aquifers are saturated underground layers that store
and transmit groundwater. These layers can be composed of loose material like gravel and sand, or solid rock like shale or limestone. In loose material (also called “unconsolidated material”), groundwater flows through the pore space (fig. 1a). In solid rock (also called “consolidated material”), groundwater flows through the cracks and fractures in the rock (fig. 1b). Groundwater flows quickly through coarsegrained materials, like sand or gravel. It flows more slowly in fine-grained materials, like clay or silt. In consolidated aquifers, water flows more quickly in places where there are many fractures.
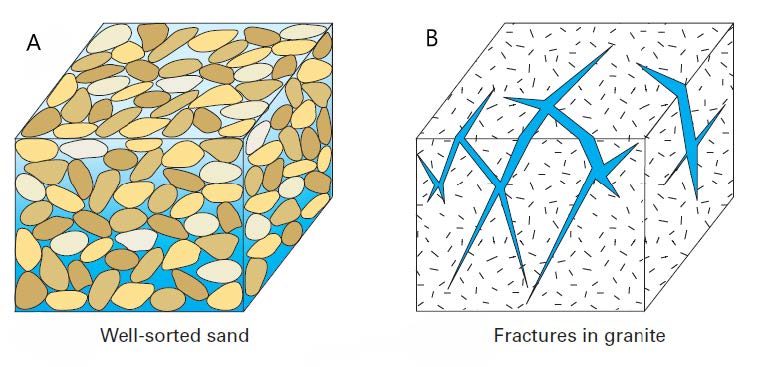
Most of the groundwater pumped for human use comes from aquifers that are made up of coarse unconsolidated materials or highly fractured rock. Underground layers of materials that tend to block or slow down the movement of water, like clay, are referred to as confining layers or aquitards. When there is no confining layer between an aquifer and the ground surface, the aquifer is considered “unconfined.” These aquifers are often located at shallow depths below ground surface. Aquifers that are below a confining layer are called “confined” aquifers and these are often located deep below the ground surface.
Groundwater is often thought of as a separate resource from surface water, like rivers and lakes. However, these water bodies are all connected. Figure 2 shows some typical ways that groundwater and surface water interact. When rain falls on the land, some of it will run off into surface water, some will be taken up by plants, and some will flow down through the soil. This process is called “recharge,” and it provides water for the aquifers below. Groundwater flows below ground, sometimes returning to the surface to supply water for a stream or river. In other cases, shallow groundwater flows downwards, recharging a deeper aquifer. When a pump is used to remove groundwater from an aquifer, this causes additional movement of water below ground towards the area being pumped.
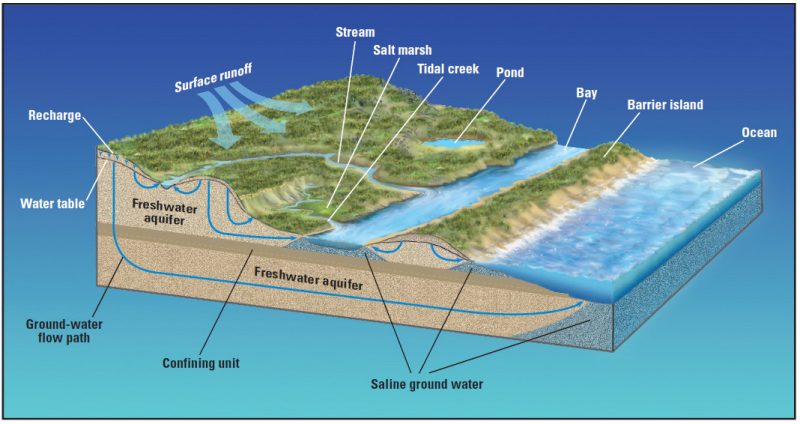
Groundwater in Virginia
Virginia’s diverse geography means that the amount of available groundwater can be very different across the state. There are five physiographic provinces within Virginia (fig. 3). Physiographic provinces are geographic regions with similar topography, geologic features, and subsurface rock types. The differences between these provinces influence the quantity of groundwater available. This is described in Table 1, summarized from the Virginia State Water Resources Plan (VDEQ 2015).
Province |
Typical features |
Impact on groundwater quantity |
Impact on groundwater quality |
---|---|---|---|
Coastal Plain |
Flat landscape with wide tidal rivers, barrier islands, and the Eastern Shore. The only province in the state where aquifers are composed of unconsolidated aquifers consisting of layers of sand, gravel, shell rock, silt, and clay. |
Yields are generally high. However, high pumping rates from deep confned aquifers with not enough recharge has resulted in land subsidence in some areas. |
Saltwater intrusion can occur in wells close to coast. Pollution in shallow unconfned aquifers can occur from urban and agricultural activities. Otherwise, quality is generally good. |
Piedmont |
Rolling topography with deep bedrock. However, subsurface geology across the province is diverse. |
Highly variable. Groundwater is primarily transmitted through fractures and faults within the bedrock. Large yields are possible in regions with extensively fractured bedrock, such as the base of the Blue Ridge Mountains. |
Highly variable. Some areas have problems with high iron and acidity. |
Blue Ridge |
Mountainous region underlain by granite and metamorphic rocks that are largely impervious. |
Generally low. Groundwater movement mostly occurs in fractures and faults within the underlying bedrock, and recharge is low. However, some higher yields occur near Roanoke and the western fanks around Buena Vista. |
Some locations have high iron concentrations. |
Valley and |
Ridges are mostly sandstone, |
The valley lowlands contain |
Much of the groundwater is |
Ridge |
with shale and carbonate materials in the valleys. Deeper materials are generally consolidated sedimentary rocks. Eroded limestone has created features like springs, sinkholes, and sinking streams that recharge groundwater. |
some productive aquifers with sustained yields up to 1,000 gpm. Yields in upland ridge regions are lower, but sometimes sufcient for domestic or light rural use. |
recharged by sinking streams, which can introduce serious water quality problems because surface water often contains contaminants. Groundwater is typically hard due to carbonite formations. |
Appalachian |
A rugged landscape with |
Highly variable. Groundwater |
Generally poor. Shallow water |
Plateau |
small, narrow valleys twisting through the mountains. Subsurface primarily consists of consolidated materials, including well-cemented sandstone. |
primarily moves irregularly through features like rock fractures and coal bed cleats. |
(<100 feet below ground surface) has high acidity levels and mineral concentrations. Deep water (>300 feet bgs) is naturally saline. Quality is better between 150-300 feet bgs, but can also be acidic in coal mining areas. |
Groundwater resources can also vary significantly within provinces. Even if two wells are drilled in the same province, the amount of water they provide will depend on their specific location, depth, and quality of construction. However, geographic provinces can provide some general insights into available groundwater resources.
In many parts of the state, groundwater exists mostly in consolidated material like limestone, granite, and shale. In these locations, groundwater flow is highly variable and depends on the presence of fractures and faults. A well drilled in highly fractured material will usually produce more water than a well drilled in material with few fractures. However, the presence of fractures can vary a lot over small areas, making it hard to predict how much water a well can yield. For instance, wells in the Piedmont region can produce anywhere from less than 1 gallon per minute to over 1,000 gpm (VDEQ 2015). In these regions, site-specific evaluation by a professional will likely be needed to determine if productive groundwater pumping is possible.
The Coastal Plain is the only region of the state with mostly unconsolidated aquifer material. Aquifers in this region are made of coarse material that can often support high pumping rates. However, many of the most productive aquifers in this region (for instance, the Potomac Aquifer) are deep underground below confining layers. This slows down recharge into the aquifer, so not enough water flows into the aquifer to replace the water that is pumped out. Resulting problems include groundwater shortages, sinking land, and saltwater flowing into wells. Virginia’s Ground Water Management Act of 1992 provided a framework to create groundwater management areas (GMAs) in regions where the above issues were observed. In GMAs, the withdrawal of groundwater is subject to stricter regulation than in the rest of the state. Virginia currently has two GMAs. The Eastern Virginia Groundwater Management Area includes all areas on the mainland of the state east of I-95.
The Eastern Shore Groundwater Management Area includes Accomack and Northampton counties (fig. 3). More details on requirements for groundwater use in GMAs are provided in the section called “Regulatory Requirements.”
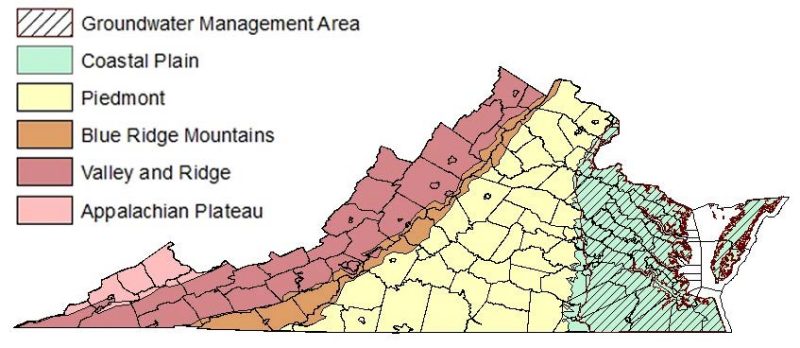
The Risks of Overuse
Groundwater is one of the largest stores of fresh water on earth. In fact, there is almost 100 times as much groundwater across the globe than there is surface water in all rivers, streams, and lakes combined.
Nevertheless, it is not an endless resource, and pumping too much groundwater in a given location can cause problems. When groundwater is pumped, water levels around the well will decline. This drop in water levels is called “drawdown.” If the pumping rate is high, then the amount of drawdown can be quite severe and impact a very large area. This is especially true when there are many wells removing water in the same area.
This is the situation that’s occurred at the Ogallala Aquifer that lies underneath eight states in the Midwest. Since pumping began to support irrigation, groundwater levels have dropped by over 150 feet in some areas. Many farmers have had to abandon their wells and drill new ones because the water level fell below the bottom of their well. Even if a well can still be used, it requires more energy (and more money) to pump water from deeper levels. Between 4 to 6 feet of water were being removed from the aquifer each year, but only one-half inch was flowing back in. This slow recharge occurred because the water in the Ogallala Aquifer is very old – by some estimates, over 3 million years old (Braxton Little 2009).
Groundwater pumped from deep, confined aquifers can take centuries or even longer to fill back up again. Shallow, surficial aquifers are often replenished much more quickly than deep groundwater located below confining layers (fig. 4).
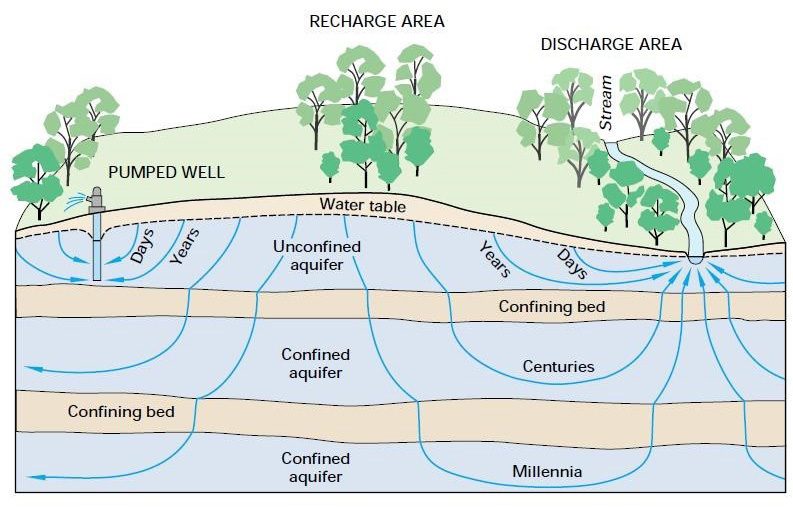
Groundwater pumping can also cause land subsidence. Removing water from an aquifer can cause the gravel and sand in the aquifer to become more compact.
This causes the land surface above the aquifer to drop. Compaction and land subsidence is typically a slow process. It can continue years or even decades after groundwater pumping stops, as the land surface “catches up” to the new, lower pressure in the aquifer. A well-known example of land subsidence from groundwater pumping is in California’s San Joaquin valley, where land elevations have declined by over 30 feet (fig. 5).
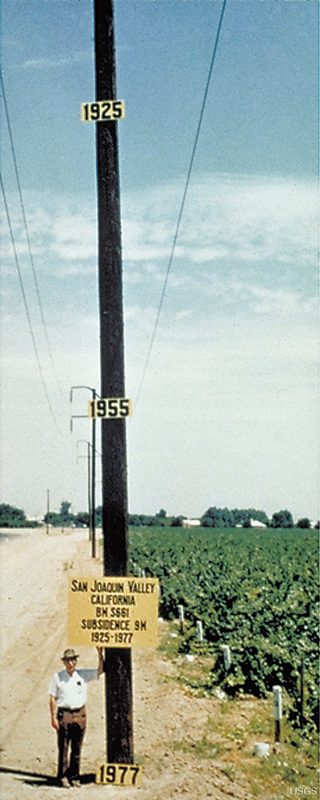
Groundwater pumping near the coast can also lead to saltwater intrusion. Because saltwater is denser than fresh water, fresh groundwater floats on top of underlying saline groundwater in areas near the ocean. Under natural conditions, the zones where fresh groundwater and saline groundwater mix stay very near the coast or far below the land surface.
When pumping results in drawdown, this can cause saltwater to be drawn closer towards the pumped area. This increases the concentration of salt in the pumped well water. If salt concentrations become too high, wells can no longer be used for drinking water or agriculture. For instance, more than 120 drinking water supply wells in Cape May County, New Jersey, have been abandoned due to saltwater intrusion (USGS 2000a).
Here in Virginia, groundwater use hasn’t yet created problems as dramatic as those in California or the Midwest. However, these examples show the risks that could occur if groundwater isn’t well managed. In the Hampton Roads area, land elevations have declined by about 2 meters since the 1940s (Eggleston and Pope 2013). This has largely been caused by groundwater pumping. The flat topography of the Tidewater area means that even small changes in land elevation can increase flood risk. Declines in water levels can also increase the risk of wells going dry during droughts, which is when the need for water is the greatest.
This is what happened during the 1999-2002 drought in Virginia, when thousands of private wells failed (VDEQ 2015). Finally, rising concentrations of salt have been observed in some wells on the Eastern Shore (VDEQ 2013). This suggests that saltwater intrusion is already occurring in some places. The state’s groundwater must be proactively managed to keep these problems from getting worse. This will help ensure that all users will have sufficient high-quality water long into the future.
Regulatory Requirements
Disclaimer: This information is current and accurate as of the time of publication of this bulletin (2023).
However, readers are advised to contact the Virginia Department of Environmental Quality (VDEQ) Office of Water Supply, https://www.deq.virginia.gov/water/water-quantity, to access the most up-to-date information about regulatory requirements in Virginia.
Two regulations in Virginia relate to using groundwater for irrigation. The first is the Virginia Water Withdrawal Reporting (VWWR) Requirement (9VAC25-200-10). This is a statewide requirement that applies to any person or organization withdrawing over 1 million gallons of water for crop irrigation in any month. This is approximately equal to the amount of water needed to apply 1 inch of water per week to a 9-acre field. The rule also applies to water users that withdraw water for other purposes (including livestock watering) if they use more than 10,000 gallons per day. This regulation requires that water users report monthly water withdrawals to the Virginia Water Use Data System. This gives planners a more accurate estimate of water usage across the state. These withdrawal reports must be measured using a flow meter installed near the withdrawal point or another approved methodology. Approved methodologies are described in the VDEQ Instructions for Estimating Raw Water Irrigation (link is provided in “Additional Resources” section, below).
The second requirement is based on the Ground Water Management Act of 1992 (Code of Virginia Title 62.1, Chapter 25). This requirement only applies to water users within a GMA who withdraw more than 300,000 gallons in any month. This is approximately equal to the amount of water needed to apply 1 inch of water per week to a 2.75-acre field. These users must obtain a groundwater withdrawal permit. The first step of the permitting process is a pre-application meeting between the applicant and VDEQ permit writers at the Office of Water Supply. During this meeting, applicants can discuss their water needs, and VDEQ staff explain what is needed to obtain a permit. This also provides an opportunity to identify potential problems early on, before going through a lengthy permitting process. For instance, if the applicant wants to withdraw large volumes of water in a location where groundwater supplies are already very stressed, this can be addressed early to avoid having a permit denied further along in the process.
Following the meeting, the user submits a permit application. This application typically includes the following information:
A demonstration of need for the proposed amount of water.
An evaluation of alternative water supply sources.
An evaluation of water quality needed for application.
A geophysical log (typically completed by well driller prior to installing the well).
A well completion form that describes how the well was constructed (typically completed by well driller).
Information on aquifer properties (testing is generally done during well construction).
A plan to mitigate impacts to other nearby groundwater users. This document is aimed at avoiding conflicts between water users.
A water conservation and management plan. This could include information on the use of water-saving devices, efficient irrigation practices, leak detection and repair, evaluation of potential water reuse options, and use reduction during water shortage emergencies.
Ideally, the permitting process will include regular communication between the applicant and VDEQ. The process of obtaining a permit also generally requires cooperation between VDEQ and the well driller. This will help ensure that all materials for a permit are collected during well drilling and installation and can help avoid problems that could result in a permit being denied. If a permit application is submitted without all the necessary information, VDEQ personnel will generally work with the applicant to obtain the missing information. Agricultural users are exempt from the permit application fee, which ranges from $1,200 to $6,000 for other types of users.
After an application is submitted, VDEQ staff reviews the application and conducts a technical evaluation to assess how the withdrawal will impact the quantity and quality of groundwater in nearby areas. A 30-day public notice period is required before any new permit can be issued. Permit holders are also required to report how much water they use on a quarterly basis. Holding a permit is important for water users to avoid fines associated with noncompliance. Furthermore, it can provide some protection from the impacts of other permitted and unpermitted users and reduces liability for the owner if the new well negatively impacts neighboring wells. Finally, it provides a record of water use for future permitting and regulatory changes.
Well Drilling and Maintenance
It is important that groundwater wells are drilled, constructed, and maintained properly. This helps ensure that the well is productive and does not introduce contamination into groundwater. Inefficient well design and improperly sized pumps can result in high pumping costs and reduce the well’s functional lifespan. To avoid this, wells should always be drilled and constructed by an experienced, licensed well driller. A diagram for typical well construction in unconsolidated materials (such as those that occur in the Coastal Plain) is shown in figure 6. After a borehole is drilled, a well casing and screen is inserted into the borehole. The screen is a portion of the casing with slots or openings where the water can flow through. The screen is surrounded by a filter pack made of sand, gravel, or both. This helps filter out fine material from the surrounding aquifer and prevent it from entering the well. After the filter pack is set, grout is pumped in the space between the well casing and borehole wall. This prevents contamination from moving between aquifers, or from the ground surface into the aquifers below.
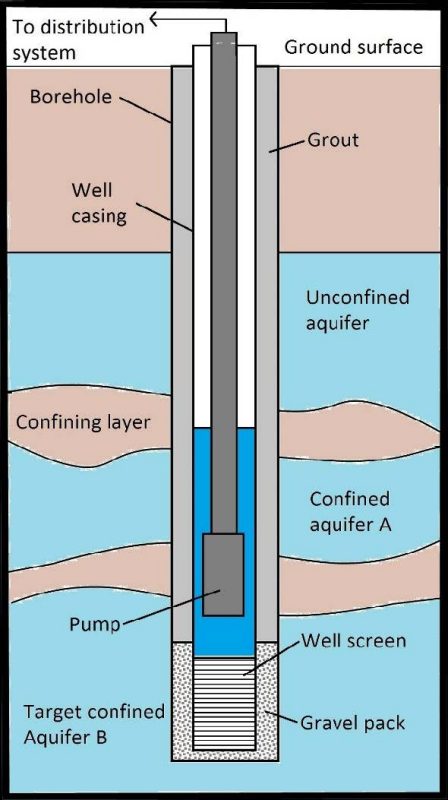
After the well is constructed, it should be developed. Well development uses pumping and other physical methods to remove fine materials and drilling fluid from the well and filter pack. This will improve the well’s productivity by allowing water to flow into the well more quickly and preventing sand and silt from being drawn into the pump.
Wells drilled into bedrock are often constructed differently. After drilling a borehole, the well casing is inserted to the top of the bedrock, and grout is pumped into the space between the well casing and the borehole walls. However, installing a screen and filter pack is not always necessary for wells drilled into consolidated material.
It is important that well construction material be chosen based on the surrounding aquifer materials and water quality. For instance, carbon steel screens are more susceptible to corrosion than stainless steel or PVC. This means they may quickly corrode in places where groundwater is acidic or saline. If the screen slots and filter pack material are too coarse, or if no filter pack is used, sand and silt from the surrounding aquifer can flow into the well. This material can settle in the bottom of the well or be pulled into the pump. Abrasion from pumping sand is one of the most common causes of pump failure. It can also damage irrigation lines and sprinklers.
Ideally, a pumping test should be conducted to determine the amount of drawdown that will occur for a given pumping rate. This will help determine how much water can be sustainably pumped from the well and what size pump is necessary. An undersized pump will not provide water at the necessary flow rate or pressure. An oversized pump will cost more and have to be throttled back, increasing pumping costs. A flow meter and pressure gauge should be installed with the well so that the irrigator can monitor flow rates and operating pressures. This will make it easier to identify problems with the pump, manage and schedule irrigation more efficiently, and assist in reporting water usage (if required). Proper well installation, construction and testing can result in significant savings in long-term operation and maintenance costs.
Just like any other type of equipment, maintenance is important in keeping wells functioning as they age. Through time, screen openings can become plugged or covered with silt or clay, bacteria, or mineral deposits. Any type of blockage will slow water flow into the well. This lowers the water level within the well, meaning the pump must exert more energy to bring the water to surface. This increases pumping costs, and pumps can become damaged if the water level drops below their intake. When blockages become severe, a well will have to be rehabilitated. This typically requires specialized tools, chemicals, and expertise. Well rehabilitation should always be done by a licensed well driller. Regularly measuring pump flow rates and the static (non-pumping) water level within the well can be useful in identifying problems early. This is important because delaying well rehabilitation can increase costs or make rehabilitation impossible.
The water level in the well should not drop below the pump intake (which could damage the pump) or the top of the screen (which can accelerate mineral buildup and blockage). Annual chlorination can help prevent the growth of bacteria that cause biological blockage. However, this process can be challenging to implement and ineffective if not done correctly.
Irrigators interested in shock chlorination should review Virginia Household Water Quality Program: Shock Chlorination: Disinfecting Private Household Water Supply Systems, Virginia Cooperative Extension publication 442-663 (Benham and Ling 2011).
Estimating Groundwater Needs
In regions where groundwater yields may be variable or low, it is important to confirm that a well will be able to provide enough water to meet irrigation needs.
The amount of water required from an irrigation well is determined by crop water requirements and the irrigation system itself. Crop water requirements vary from year to year based on the amount of rainfall that occurs during the growing season. It is best to determine water needs based on a dry year, since this is when irrigation is most critical. To calculate the volume of water needed to meet crop water requirement, you can multiply the water requirement (in inches) by the area of the field to be irrigated. For example, peak water requirements for corn are about 0.3 inches of water per day (Kranz et al. 2008). Applying 1 inch of water to a 1-acre field requires 27,154 gallons of water (this volume of water is referred to as an “acre-inch”). This means that applying 0.3 inches of water to a 10-acre field will require about 81,000 gallons per day:

To calculate the minimum flow rate needed to provide this volume of water, you can divide the volume of water by the time that the irrigation system will be operating. For example, if an irrigation system is operated for 8 hours over the course of a day, it will require a pumping rate of 170 gallons per minute to provide 81,462 gallons per day:

If your operation is in a region where groundwater availability is highly variable (such as the Piedmont), it may be useful to consult with a licensed hydrogeologist to find out if a well will be able to meet your water requirements.
Conclusions
Irrigation with groundwater can be a reliable method for managing drought risk. However, it can be costly in terms of both money and time. Proper well construction pays off in the long run by reducing the chances of water shortages and irrigation system damage. It is important to comply with groundwater permitting and reporting requirements to avoid fines. Because groundwater resources vary significantly between and within provinces throughout the state, producers interested in groundwater irrigation should first determine whether a well will be able to meet their requirements. Consulting with a licensed hydrogeologist could be useful. This also ensures that there is a record of your water use in case regulations change. Groundwater is not a limitless resource, but responsible water use helps ensure that enough groundwater will be available for Virginians long into the future.
Additional Resources
Well Construction and Maintenance
Boman, B., S. Shuklan, and J.D. Hardin. 2006. “Design and Construction of Screened Wells for Agricultural Irrigation Systems.” University of Florida Institute of Food and Agricultural Sciences Extension Circular 1454. http://edis.ifas.ufl.edu/ch166.
Haman, D.Z. and G.A. Clark. 1998. “Water Wells for Florida Irrigation Systems.” University of Florida Institute of Food and Agricultural Sciences Extension Circular 803. http://edis.ifas.ufl.edu/wi002.
Scherer, T. 2013. “Care and Maintenance of Irrigation Wells.” North Dakota State University Extension Service Publication AE-97 (Revised). https://www.ag.ndsu.edu/publications/crops/care-and-maintenance-of-irrigation-wells.
Swistock, B. 2022. “Water Facts #32: Water Well Maintenance and Rehabilitation.” Penn State University Extension. https://extension.psu.edu/water-well-maintenance-and-rehabilitation.
Permitting and Reporting Requirements in Virginia
VDEQ. 2021. “Decision Tree for Agricultural Water Withdrawal Reporting.” https://www.deq.virginia.gov/home/showpublisheddocument/2123/637703205572900000
VDEQ. 2023. Groundwater Withdrawal Permit (GWP) Manual." https://www.deq.virginia.gov/home/showpublisheddocument/17517/638150874579470000
VDEQ. 2023. “Groundwater Withdrawal Permitting Program Overview.” https://www.deq.virginia.gov/permits/water/water-withdrawal/ground-water
References
Barlow, P.M. 2003. “Ground Water in Freshwater-Saltwater Environments of the Atlantic Coast.” U.S. Geological Survey Circular 1262. https://pubs.usgs.gov/circ/2003/circ1262/.
Benham, B., and E. Ling. 2011. “Virginia Household Water Quality Program: Shock Chlorination: Disinfecting Private Household Water Supply Systems.” Virginia Cooperative Extension publication 442-663. https://www.pubs.ext.vt.edu/442/442-663/442-663.html.
Braxton Little, J. 2009. “The Ogallala Aquifer: Saving a Vital U.S. Water Source.” Scientific American, March 1, 2009. https://www.scientificamerican.com/article/the-ogallala-aquifer/.
Eggleston, J., and J. Pope. 2013. “Land Subsidence and Relative Sea-Level Rise in the Southern Chesapeake Bay Region.” USGS Circular 1392. https://pubs.usgs.gov/circ/1392/pdf/circ1392.pdf.
Kranz, W.L., S. Irmak, S.J. van Donk, C.D. Yonts, and D.L. Martin. 2008. “Irrigation Management for Corn.” University of Nebraska – Lincoln Extension Bulletin G1850. http://extensionpublications.unl.edu/assets/pdf g1850.pdf.
U.S. Geological Survey (USGS). 2000a. “Ground-water Resources for the Future – Atlantic Coastal Zone.” Fact Sheet 085-00. https://water.usgs.gov/ogw/pubs/fs00085/pdf/fs-085-00.pdf.
USGS. 2000b.: “Ground-water Resources for the Future – Land Subsidence in the United States.” Fact Sheet 165-00. https://water.usgs.gov/ogw/pubs/fs00165/SubsidenceFS.v7.PDF.
Virginia Department of Environmental Quality (VDEQ). 2013. “Ambient Groundwater Quality Monitoring Strategy.”
VDEQ. 2014. “Factsheet: Groundwater Well Installation in Groundwater Management Areas.”
VDEQ. 2015. “Virginia State Water Resources Plan.” https://www.deq.virginia.gov/home/showpublisheddocument/8227/637534969441500000.
Winter, T.C., J.W. Harvey, O.L. Franke, and W.M. Alley. 1998. “Ground Water and Surface Water – A Single Resource.” USGS Circular 1139. https://pubs.usgs.gov/circ/circ1139/.
Virginia Cooperative Extension materials are available for public use, reprint, or citation without further permission, provided the use includes credit to the author and to Virginia Cooperative Extension, Virginia Tech, and Virginia State University.
Virginia Cooperative Extension is a partnership of Virginia Tech, Virginia State University, the U.S. Department of Agriculture, and local governments. Its programs and employment are open to all, regardless of age, color, disability, sex (including pregnancy), gender, gender identity, gender expression, genetic information, ethnicity or national origin, political affiliation, race, religion, sexual orientation, or military status, or any other basis protected by law.
Publication Date
June 22, 2023