How Is Ozone Gas Used To Process Foods?
ID
FST-356P

Background
Ozone (O3) is a chemical compound made up of three oxygen molecules. Since the late 1800s, ozone has historically been used to disinfect drinking water (Tiwari and Rice 2012). More recently, it has evolved to have new uses in the food industry as a food processing method. Ozone is a colorless gas commonly known as the thick layer covering Earth and protecting us from the sun’s rays. It is used to kill bacteria in various types of food (e.g., fruits, vegetables, spices, meats, seafood); to sanitize food equipment, food packaging, and contact surfaces; to reduce undesirable odors; and to extend a food’s shelf life (O’Donnell et al. 2012). Ozone can be applied either as a gas or dissolved in water as an aqueous treatment (Brodowska, Nowak, and Smigielski 2018; Miller, Silva, and Brandão 2013; O’Donnell et al. 2012). Figure 1 shows a glass container filled with tomatoes that have been treated with ozone gas.
How It Works
Even though ozone is made up of oxygen, it is much less stable than the oxygen we breathe (O2). In fact, ozone’s instability is what allows it to be a safe and effective food processing technology because it decreases contamination on food without leaving any harmful chemical residue. Ozone is made up of three oxygen molecules; however, oxygen prefers to be in pairs (two O molecules). Therefore, the third molecule easily separates from the pair into what is known as a free radical (a solo molecule). This free radical searches for a compatible pair. As a result, the free radical attaches to other molecules on biological surfaces and breaks the surface molecules apart, thereby destroying microorganisms (Brodowska, Nowak, and Smigielski 2018).
Technology
Because ozone degrades rapidly, it must be generated immediately before use. The two most common methods of generating ozone are by (1) corona discharge (CD) and/or (2) UV photochemical generation. Ozone is produced with CD technology by passing dry air — or an O2-containing gas mixture — through a temperature-controlled high-energy electrical charge/field. Ozone is generated with UV photochemical generation by shining UV light (140-190 nm) on O2 molecules. In both methods, O2 molecules are split into individual oxygen (O) atoms that join with some other floating O2 molecules to form ozone (O3); (Brodowska, Nowak, and Smigielski 2018).
Once created, ozone can be applied directly as a gas or dissolved into water as part of an aqueous solution. Figures 2 and 3 show examples of ways that ozone is created in a laboratory setting. Ozone is more effective when applied as a gas than when dissolved in water. In water, ozone dissociates (breaks apart) quickly to become oxygen (Miller, Silva, and Brandão 2013).
Creating ozone using CD technology has advantages: a lot of ozone can be created, it can be used in water and many other applications, it removes odor compounds, and it is great for long-term equipment use. Creating ozone with UV technology, while cheaper, yields less ozone with limited applications (Brodowska, Nowak, and Smigielski 2018).
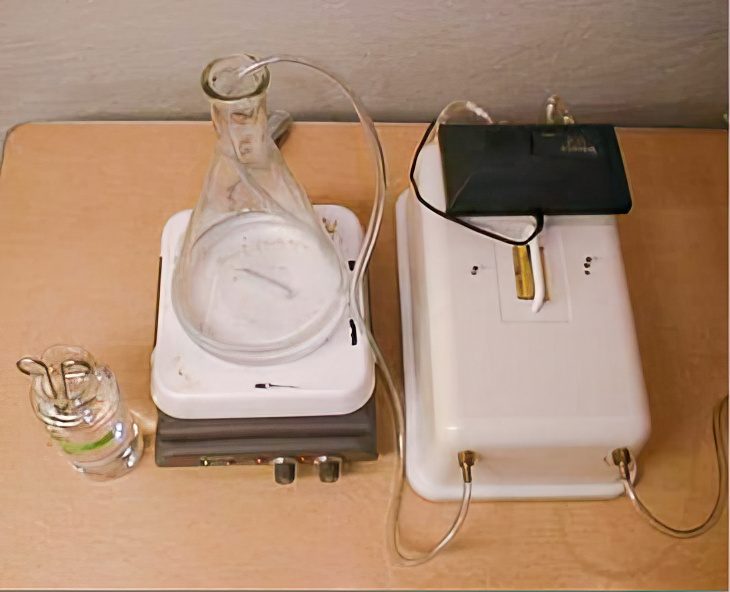
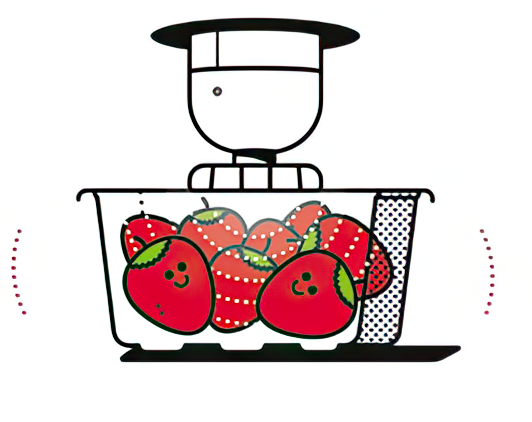
Efficacy
Ozone is 50% stronger than chlorine without using as high of a concentration. Leftover ozone quickly decomposes into oxygen, leaving no residue in foods. Ozone has been proven effective for enhancing microbial safety and extending the shelf life of spices, produce, meats and poultry, fish, dairy, and beverages. Its effectiveness against pathogens and spoilage microorganisms depends on a variety of factors (e.g., food product, microorganisms being targeted, contamination level, gas or aqueous, concentration used, contact time, humidity [as a gas application], ambient or product temperature, etc.; (O’Donnell et al. 2012). Ozonated water applied to blueberries can reduce pathogenic E. coli O157:H7 and Salmonella by approximately 99.999% (a 5-log reduction; Bialka and Demirci 2007). Exposing apples and pears to miniscule amounts of ozone gas can result in an extended shelf life (Brodowska, Nowak, and Smigielski 2018; Skog and Chu 2001).
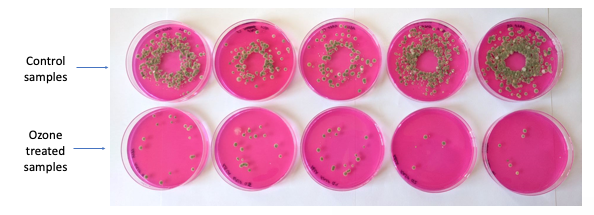
Benefits
Ozone is effective against many types of food-related microorganisms (e.g., bacteria, fungi, mold, and yeast). Additionally, ozone does not negatively affect the qualities (appearance, texture, and nutrition) of the food products it treats. It is both cost-effective and eco-friendly; it is cheaper to purchase and maintain ozone systems than to continually purchase other disinfectants (Brodowska, Nowak, and Smigielski 2018). Ozone is also used as a replacement for insecticides due to increasing environmental problems and legislations that regulate or prohibit pesticide use (O’Donnell et al. 2012).
Current Usage
In 2001, the U.S. Food & Drug Administration (2001) approved ozone as an antimicrobial for direct contact with water and foods of all types. It is also used in the food industry to sanitize/decontaminate food surfaces, food equipment, packaging materials, water used in processing, and storage equipment.
References
Bialka, K. L., and A. Demirci. 2007. “Decontamination of Escherichia coli O157:H7 and Salmonella enterica on Blueberries Using Ozone and Pulsed UV-Light.” Journal of Food Science 72 (9): M391- 96. doi:10.13031/2013.24876.
Brodowska, A. J., A. Nowak, and K. Śmigielski. 2018. “Ozone in the Food Industry: Principles of Ozone Treatment, Mechanisms of Action, and Applications: An Overview.” Critical Reviews in Food Science and Nutrition 58 (13): 2176-201. doi:10.1080/10408 398.2017.1308313.
Miller, F. A., C. L. M. Silva, and T. R. S. Brandão. 2013. “A Review on Ozone-Based Treatments for Fruit and Vegetables Preservation.” Food Engineering Reviews 5 (2): 77-106. doi:10.1007/s12393-013-9064-5.
O’Donnell, C., B. K. Tiwari, P. J. Cullen, and R. G. Rice. 2012. “Status and Trends of Ozone in Food Processing.” In Ozone in Food Processing, edited by C. O’Donnell, B. K. Tiware, P. J. Cullen and R. G. Rice, 1-5. West Sussex, UK: Wiley-Blackwell.
Skog, L. J., and C. L. Chu. 2001. “Effect of Ozone on Qualities of Fruits and Vegetables in Cold Storage.” Canadian Journal of Plant Science 81 (4): 773-78. doi:10.4141/p00-110.
Tiwari, B. K., and R. G. Rice. 2012. “Regulatory and Legislative Issues.” In Ozone in Food Processing, edited by C. O’Donnell, B. K. Tiware, P. J. Cullen and R. G. Rice, 7-17. West Sussex, UK: Wiley-Blackwell.
U.S. Food and Drug Administration. 2001. “Part 173 – Secondary Direct Food Additives Permitted in Food for Human Consumption.” Federal Register 66 (123): 33830. www.govinfo.gov/content/pkg/FR-2001-06-26/pdf/01-15747.pdf.
Wang, L., X. Fan., K. Sokorai., and J. Sites. 2019. “Quality Deterioration of Grape Tomato Fruit During Storage After Treatments With Gaseous Ozone at Conditions That Significantly Reduced Populations of Salmonella on Stem Scar and Smooth Surface.” Food Control 103:9-20.
Acknowledgements
This work is supported by the Agriculture and Food Research Initiative competitive grant program A4131 (grant No. 2015-69003-23410/project accession No. 1005440, “Enhancing the Safety and Quality of Fresh Produce and Low-Moisture Foods by Waterless Non-thermal Technologies”) from the USDA National Institute of Food and Agriculture.
Virginia Cooperative Extension materials are available for public use, reprint, or citation without further permission, provided the use includes credit to the author and to Virginia Cooperative Extension, Virginia Tech, and Virginia State University.
Virginia Cooperative Extension is a partnership of Virginia Tech, Virginia State University, the U.S. Department of Agriculture, and local governments. Its programs and employment are open to all, regardless of age, color, disability, sex (including pregnancy), gender, gender identity, gender expression, genetic information, ethnicity or national origin, political affiliation, race, religion, sexual orientation, or military status, or any other basis protected by law.
Publication Date
May 20, 2020