Basic Tomato (Lycopersicon esculentum) Physiology and Morphology
ID
SPES-508NP
Economic Importance
Tomatoes are a globally significant crop with extensive cultivation worldwide. In the United States, tomato production ranks fourth on a global scale. The state of Virginia harvested between 2,200 and 2,800 acres of fresh-market tomatoes per year, with an average yield of 25 tons/acre, and nearly $25 million in value in 2018, with most of the fruit intended for wholesale markets and distributed across the east coast of the U.S. (Virginia Department of Agriculture and Consumer Services, 2020). Understanding the plant functions, anatomy, and reactions to abiotic stressors is crucial for achieving the desired crop outcomes and optimizing yield within the current production system in Virginia.
History
The tomato plant is native to western South America, specifically the region encompassing present-day Ecuador, Peru, Bolivia, and northern Chile. The plant's wild ancestors, small and bitter berries, were first cultivated by indigenous peoples in these areas. The earliest evidence of tomato cultivation dates to around 500 BC in what is now modern-day Mexico. The Mesoamerican civilizations, including the Aztecs and Mayans, were among the first to cultivate and consume tomatoes. They appreciated the tomato's vibrant colors and incorporated it into their cuisine (Bergougnoux, V. 2014; Smith, 2001).
European exploration brought tomatoes to the attention of the Western world. Spanish conquistadors encountered tomatoes during their expeditions to the Americas and introduced them to Europe. Initially, Europeans regarded tomatoes with suspicion due to their similarity to poisonous plants from the same family, such as belladonna. In Europe, tomatoes were initially cultivated as ornamental plants. It was believed that they possessed aphrodisiac properties, and the fruit's vibrant colors made it an attractive addition to gardens. However, tomatoes began to gain culinary popularity gradually. By the 17th and 18th centuries, tomatoes were used in various European cuisines, particularly Italy and Spain (Bergougnoux, V. 2014; Smith, 2001).
Tomatoes arrived in North America during the 18th century. Thomas Jefferson grew tomatoes at his Monticello estate in Virginia, helping to popularize their cultivation in the country. However, tomatoes were still met with skepticism in some parts of North America, with some considering them to be poisonous or unfit for consumption. Advances in scientific understanding helped dispel myths about their toxicity. The discovery that tomatoes belonged to the nightshade family, which includes many edible plants, reassured people about their safety.
Anatomy and Morphology
Tomatoes are dicot plants structured of various anatomical organs that contribute to their growth and development. Normally, the tomato plant has a taproot system with a main primary root that extends vertically into the soil. From the primary root, lateral roots branch out, spreading horizontally to absorb water and nutrients. However, tomato plants can have either a fibrous or a taproot, depending on the tissue they are growing from (Ouyang et al., 2021). If the plant comes from a seed, the main roots will be a taproot; whereas when plants grow from a vegetative part, the roots will be fibrous. About 60% of tomato roots are 11 inches below the soil
(Heuvelink, 2018). The roots play a crucial role in anchoring the plant and absorbing essential elements for growth. The basic root develops from the embryonic radicle. The taproot grows vertically into the soil, providing stability to the plant and serving as the primary channel for water and nutrient uptake. Lateral roots are thinner and extend horizontally in the soil. They arise from the pericycle, a layer of cells in the root's vascular cylinder (stele). These lateral roots increase the root surface area, enhancing the plant's ability to absorb water and nutrients.
At the microscopic level, the root surface is covered with tiny root hairs (Figure 1). Root hairs are elongated, finger-like projections that emerge from the epidermal cells of the root. They greatly increase the surface area available for water and nutrient absorption. Root hairs play a crucial role in facilitating the uptake of water and mineral ions from the soil. Root hair densities in tomato plants range from approximately 100 to 500 root hairs per square millimeter of root surface area. These numbers may vary depending on the specific tomato variety, growth stage, and the region of the root being examined (e.g., root tip or mature sections).
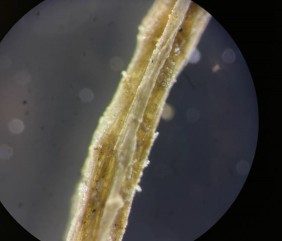
The tip of the tomato root is covered by a protective structure called the root cap. The root cap is composed of specialized cells that provide protection to the root as it grows through the soil or growing media. It also secretes a slimy substance called mucilage, which helps lubricate the root's passage through the soil and aids in water absorption.
The stem of a tomato plant is characterized by its herbaceous nature. It grows erect and provides support to the plant. The stem consists of nodes and internodes. Nodes are the points along the stem where leaves, lateral branches, and flowers emerge. Internodes are the spaces between nodes and contribute to the overall length of the stem. Adventitious roots can develop from the stem, particularly when it meets the soil. The stem surface of a tomato plant is smooth and covered with tiny, fine hairs (Figure 2). These hairs, known as trichomes, can vary in density and provide protection against pests, reduce water loss, and contribute to the overall texture of the stem.
Tomato stems have leaf axils, which are the upper angles formed between the stem and the base of the leaf petiole. It is from the leaf axils that lateral branches, flowers, and fruit clusters (inflorescences) emerge. The apical meristem is found at the growing tip of the stem, known as the apical bud or shoot tip. The apical meristem is a region of actively dividing cells responsible for the vertical growth of the stem. It produces new cells that elongate and differentiate into various tissues, allowing the stem to increase in length. Certain tomato varieties, known as stoloniferous or trailing types, produce long, slender stems called stolons, which grow along the ground, and at their nodes, they can form adventitious roots, leading to the development of new plants.
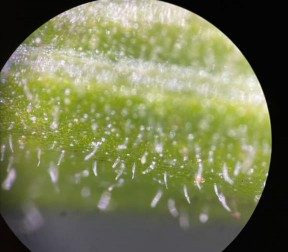
Commercial tomato plants can be divided into two categories based on their stem-growing habits: indeterminate and determinate (Kandel et al., 2020). The determinate plant will decrease its vegetative development once the reproductive organs (flowers) are developed. On the other hand, indeterminate cultivars will maintain a constant growth of their stem and leaves, even after the first few flowers appear in the plant.
Tomato plants have compound leaves that are arranged alternately along the stem. Each leaf typically consists of several leaflets. The leaflets are usually ovate or lanceolate in shape, and they have a serrated margin. The leaves are green and contain chlorophyll, allowing them to carry out photosynthesis, producing energy for the plant.
The leaf blade, also known as the lamina, is the leaf’s main, flat, expanded portion. Tomato leaf blades are typically broad, ovate, or lanceolate in shape, with a smooth margin. The size and shape of the leaf blade may vary depending on the tomato variety. Tomato leaves have a network of veins running through the leaf blade (Figure 3). These veins consist of vascular tissues, including xylem and phloem, that transport water, minerals, and sugars within the leaf. The major veins, called primary veins or midribs, extend from the leaf base towards the leaf tip, branching into secondary veins and further into smaller tertiary veins.
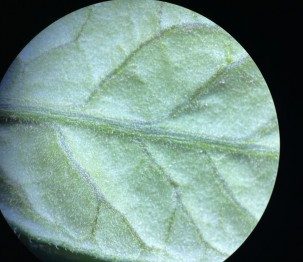
The leaf petiole is the slender stalk that attaches the leaf blade to the stem. Tomato leaf petioles can vary in length, depending on the specific tomato variety. The petiole provides support and allows for flexibility and movement of the leaf blade. The leaf axil is the upper angle formed between the petiole and the stem. In tomato plants, the leaf axils are important sites for lateral branch development, flower formation, and fruit clusters (inflorescences).
The surface of tomato leaves is typically smooth and covered with tiny, fine hairs called trichomes (Figure 3). Trichomes can vary in density and length, and they contribute to leaf texture. They can provide protection against pests, reduce water loss through transpiration, and affect leaf temperature regulation.
Tomato leaves often have small, leaf-like appendages called stipules at the base of the petiole. Stipules can vary in size and shape, ranging from tiny bristles to leafy structures. In some tomato varieties, stipules may be absent or very small and inconspicuous. On average, tomato leaves have a stomatal density ranging from approximately 50 to 150 stomata per square millimeter (mm²) of leaf surface area (Figure 4). However, it's important to note that these values can vary among different tomato varieties and even within different regions of the same leaf. Stomatal density tends to be higher on the lower (abaxial) surface of the tomato leaf compared to the upper (adaxial) surface. The lower surface is more exposed to light and involved in gas exchange and transpiration.
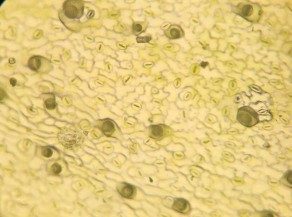
Tomato plants produce yellow, five-petaled flowers that are typically self-fertilizing (Figure 5). The flowers arise from the leaf axils, where the leaves join the stem. Each flower has both male and female reproductive organs. The male reproductive organ is the stamen, which consists of a filament and an anther containing pollen. The female reproductive organ is the pistil, which consists of the stigma, style, and ovary (Went, 1944).
Tomato flowers are typically composed of several distinct parts. At the base of the flower, there is a modified stem called the pedicel or flower stalk, which attaches the flower to the main stem of the plant. Flowers have five green, leaf-like sepals, collectively known as the calyx. The sepals enclose and protect the developing flower bud before it opens. They can be seen as small, pointed structures at the base of the flower. Flowers have five fused petals, forming a trumpet-shaped structure called the corolla. The petals are typically yellow in color, although certain varieties may have petals in different shades, such as white or pink.
The stamens are the male reproductive organs of the flower. Tomato flowers usually have five stamens, consisting of a filament and an anther. The filament is a slender stalk-like structure that supports the anther, which contains pollen grains. The pollen carries the male gametes required for fertilization. The pistil is the female reproductive organ of the flower, located in the center of the flower, and consists of three main parts: Stigma, Style, and Ovary.
The stigma is the receptive surface at the top of the pistil. It is often sticky or hairy, enabling it to capture and hold pollen grains. The style is a slender tube-like structure that connects the stigma to the ovary. It provides a pathway for pollen tubes to reach the ovary during fertilization. The ovary is the enlarged basal part of the pistil. It contains one or more ovules, which are potential seeds. After fertilization, the ovary develops into the fruit of the tomato plant.
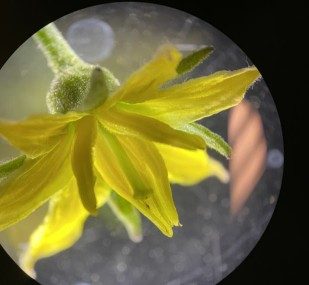
Tomato flowers are borne in clusters called inflorescences. The inflorescence of a tomato plant is called a raceme, which is a type of unbranched, elongated cluster. It consists of multiple flowers attached to a main axis or peduncle.
The fruit of a tomato plant develops from the fertilized ovary after successful pollination. It is a fleshy, berry-like structure that varies in size, shape, and color depending on the tomato variety. The fruit typically contains multiple chambers filled with seeds embedded in a gelatinous pulp. The seeds are dispersed when the fruit ripens, and the pulp becomes soft (Brewer et al., 2007). They have a fleshy pericarp, which is the wall of the fruit, surrounding the seeds. The pericarp is divided into three main layers: the exocarp (outermost layer), the mesocarp (middle layer), and the endocarp (innermost layer). The exocarp of tomato fruit is commonly referred to as the skin or peel. It is thin, smooth, and typically glossy. The color of the skin can vary widely, ranging from green (unripe) to red, yellow, orange, pink, or even striped or bi-colored, depending on the cultivar. The skin serves as a protective layer for the underlying fruit tissues.
The mesocarp or flesh of tomato fruit is the juicy, edible part. It is composed of parenchyma cells filled with water, sugars, acids, and other nutrients. The flesh is typically soft and succulent, with varying textures and flavors depending on the tomato variety. Tomato fruits contain numerous seed locules, which are the cavities or compartments within the fruit that house the seeds. The number of seed locules can vary depending on the tomato variety. Commonly, tomato fruits have multiple seed locules arranged in a radial pattern around the central core.
Each seed locule of a tomato fruit contains multiple seeds. The seeds are small, flattened, and kidney- shaped. They are encased within a gel-like substance called placental tissue. Tomato seeds are surrounded by a seed coat that protects and houses the embryonic plant. At the top of the fruit, there is a small, woody structure called the fruit stalk or pedicel. The fruit stalk connects the fruit to the peduncle, which is the main stem of the plant. The fruit stalk facilitates nutrient transport and provides support for the developing fruit (Fruit: Rančić et al., 2010).
Air Temperature
Air temperature has a significant effect on tomato plant growth and development. Different temperature ranges can influence various physiological processes and ultimately impact the overall growth and productivity of tomato plants (Heuvelink et al., 2018).
Air and soil temperature play a crucial role in seed germination and early seedling growth. The optimal germination temperature for tomato seeds typically ranges between 20°C and 30°C (68°F-86°F). Higher temperatures promote faster germination, while lower temperatures can result in delayed or uneven germination. Once germinated, tomato seedlings thrive best in slightly cooler temperatures around 18°C to 24°C (64°F-75°F).
Air temperature also affects vegetative growth in tomato plants, including leaf and stem development. Tomato plants generally prefer daytime temperatures around 21°C to 27°C (70°F-81°F) for optimal vegetative growth. In this temperature range, photosynthesis and other metabolic processes occur at an efficient rate, promoting vigorous leaf growth, stem elongation, and overall plant size.
Air temperature significantly influences flowering and fruit set in tomato plants. The ideal temperature range for successful flower development and fruit set is typically between 21°C and 24°C (70°F-75°F). Higher temperatures can inhibit pollen viability, resulting in reduced fruit sets and increased flower drops. Conversely, temperatures below 15°C (59°F) or above 32°C (90°F) can also lead to poor fruit set and decreased yield. Warmer temperatures accelerate fruit ripening, while cooler temperatures slow it down. Once the fruits have set, a temperature range of 18°C to 27°C (64°F-81°F) is generally favorable for optimal fruit development and ripening. Extreme heat or cold stress can result in uneven ripening, reduced flavor, and texture quality of the tomatoes.
Exposure to prolonged periods of extreme heat above 32°C (90°F) or extended cold spells below 10°C (50°F) can negatively impact tomato plants. High temperatures can lead to flower drop, reduced fruit quality, and increased susceptibility to pests and diseases. Cold temperatures can cause chilling injury, resulting in stunted growth, leaf damage, and reduced fruit production.
Relative Humidity
Relative humidity (the amount of moisture present in the air relative to its maximum retention capacity at a given temperature), can also have both positive and negative effects on tomato growth and yield. Relative humidity affects the rate of transpiration, which is the process by which plants lose water through their leaves. High humidity levels reduce the rate of transpiration, leading to slower water loss from tomato plants. This can be beneficial in preventing excessive water stress and maintaining adequate hydration. However, excessively high humidity for extended periods can also limit transpiration, potentially hindering water uptake and nutrient absorption.
High relative humidity levels can also create a favorable environment for the development and spread of fungal and bacterial diseases. Moist conditions provide a breeding ground for pathogens, increasing the risk of diseases such as powdery mildew, leaf spots, and fruit rot. To mitigate disease pressure, it is crucial to maintain proper air circulation, reduce leaf wetness, and manage humidity levels to minimize pathogen proliferation (Figure 6).
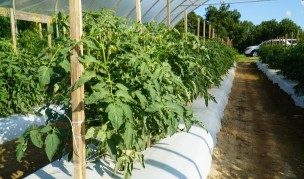
Relative humidity can influence the process of pollination and subsequent fruit set in tomatoes. High humidity, particularly when combined with high temperatures, can adversely affect pollination by reducing pollen viability and hindering pollen transfer by wind or pollinators. This can lead to poor fruit set and reduced yield. Ensuring adequate air movement and lower humidity levels during flowering can help facilitate successful pollination and fruit set.
High humidity levels during fruit development and ripening increase the risk of fruit cracking and the growth of fungal pathogens. Excessive moisture on the fruit surface can also lead to post-harvest rotting. On the other hand, very low humidity levels can cause fruit dehydration and result in reduced fruit quality, flavor, and shelf life. Overall, extremely high, or low air humidity levels can negatively impact overall plant growth and vigor. In general, a relative humidity of around 60% is considered ideal for most stages of tomato growth.
Solar Radiation
Solar radiation plays a crucial role in tomato plant growth and yield. Solar radiation is the primary energy source for photosynthesis, the process by which plants convert sunlight into energy. Tomato plants require solar radiation to produce carbohydrates, which are essential for growth and fruit development. Sufficient sunlight exposure allows tomato plants to photosynthesize efficiently, resulting in healthy foliage, increased biomass, and overall plant vigor.
The solar radiation requirement for tomato plants can be described in terms of daily light integral (DLI), which represents the total amount of photosynthetically active radiation (PAR) received by the plant over a 24-hour period. Generally, tomato plants require a DLI ranging from 15 to 30 mol/m²/day for optimal growth and fruit production. This range ensures that the plants receive sufficient light energy for photosynthesis, which is essential for plant growth, leaf expansion, flowering, and fruit development. To achieve the desired DLI, tomato plants typically thrive when exposed to direct sunlight for 6 to 8 hours per day.
Insufficient sunlight exposure can lead to tall and thin plants, reduced fruit set, and overall weaker growth. Plants respond best to a balanced spectrum of light, including both blue and red wavelengths. Blue light promotes vegetative growth and overall plant development, while red light is essential for flowering and fruiting.
Water Availability
Water is essential for maintaining plant hydration and turgidity. Sufficient water availability ensures that tomato plants can maintain proper cell turgor pressure, which is necessary for structural support and upright growth. When water is limited, plants may temporarily wilt, leading to stunted growth and reduced overall vigor.
Adequate water availability enables efficient photosynthesis, promoting the production of carbohydrates and supporting plant growth. Water is also necessary for the uptake and transport of essential nutrients from the soil to different parts of the tomato plant. Sustained water availability also supports leaf expansion and canopy development in tomato plants. Sufficient water uptake allows for the growth of large, healthy leaves, increasing the overall photosynthetic capacity of the plant. A well- developed canopy ensures maximum light interception and utilization, contributing to better growth and yield potential.
Water availability during the flowering stage is crucial for successful pollination and fruit set in tomato plants. Inadequate water supply can lead to decreased pollen viability and reduced pollination efficiency. Insufficient water during fruit development may result in incomplete fruit development, smaller fruit size, and increased susceptibility to blossom-end rot and other physiological disorders. Insufficient water during fruit development can lead to reduced fruit size, poor flavor, and increased susceptibility to cracking. Water stress during fruit ripening stages may result in uneven ripening and compromised fruit quality. Conversely, optimal water availability supports the production of well-sized, flavorful fruits, and higher yield.
Water stress also weakens the defense mechanisms of tomato plants, making them more susceptible to diseases. Drought-stressed plants are often more prone to infections by fungal pathogens, such as Fusarium and Verticillium, which can cause wilting, root rot, and other diseases. Maintaining adequate soil moisture levels reduces the risk of disease and enhances plant health.
Salinity
Salinity refers to the presence of excessive salts in the soil or irrigation water, which can have detrimental effects on tomato plant growth and yield. High soil salinity levels create an osmotic imbalance, making it more difficult for tomato plants to absorb water from the soil. This leads to water stress and reduced water uptake, affecting plant growth and development. Additionally, high salt levels can disrupt the uptake and balance of essential nutrients, such as potassium, calcium, and magnesium, further impairing plant nutrition and overall health.
Excessive salt accumulation in the root zone can result in ion toxicity, particularly from sodium (Na+) and chloride (Cl-) ions. Sodium ions can interfere with nutrient uptake and disrupt cell functioning, leading to nutrient deficiencies. Chloride ions, when taken up in high amounts, can cause leaf burn, tissue damage, and reduced photosynthetic efficiency. The imbalance of these ions affects cellular processes, metabolic activities, and overall plant performance.
Salinity stress inhibits tomato plant growth and development. Plants may exhibit stunted growth, reduced shoot, and root development, and overall decreased biomass accumulation. The impact on growth is often more severe in young plants and seedlings, as they are more sensitive to salt stress. Additionally, salinity can hinder the development of flowers, resulting in reduced fruit sets and ultimately lower yields.
High salt concentrations can cause leaf damage and chlorosis in tomato plants. Chlorosis refers to the yellowing of leaves due to a disruption in chlorophyll synthesis and functioning. Salinity- induced chlorosis can lead to reduced photosynthesis, lower carbohydrate production, and decreased energy availability for growth and fruit development.
Salinity stress negatively affects fruit quality and yield in tomatoes. The accumulation of salts in the plant can result in reduced fruit size, abnormal shape, and lower overall marketable yield. Salinity- induced stress can also affect the flavor, color, and nutritional composition of the fruits, impacting their market value and consumer acceptability.
Salinity stress weakens tomato plants, making them more susceptible to various diseases and pests. The weakened defense mechanisms and physiological imbalances caused by salinity can create favorable conditions for the growth and spread of pathogens, leading to increased disease incidence. Pests, such as nematodes, may also thrive under salinity stress and cause additional damage to the plants.
Mitigating the effects of salinity stress on tomatoes can be challenging, but several strategies can help alleviate the negative impacts. These include selecting salt-tolerant tomato varieties, improving soil drainage and leaching, implementing proper irrigation practices, and using soil amendments to reduce salt accumulation. Additionally, monitoring and managing soil salinity levels through regular testing and appropriate fertilizer application can help optimize tomato growth, yield, and overall plant health in saline environments.
Electrical conductivity (EC) is a measure of a material's ability to conduct an electric current. In the context of water or solutions, it indicates the concentration of ions or dissolved solids present in the solution, which affects its ability to conduct electricity.
For tomatoes grown in soilless or hydroponic systems, the recommended EC range is typically between 1.5 and 3.0 millisiemens per centimeter (mS/cm) during the vegetative stage. As the plants transition to the flowering and fruiting stage, the EC can be increased slightly to a range of 2.0 to 4.0 mS/cm. These values provide a good balance of nutrient availability and uptake while avoiding excessive salt accumulation.
In soil-based cultivation, the optimal EC levels can be influenced by the specific soil composition and fertility. As a general guideline, an EC range of 1.0 to 2.5 mS/cm is often recommended for tomatoes in soil. Regular soil testing and monitoring are essential to adjust and maintain the appropriate EC levels based on the specific soil conditions and nutrient requirements of the plants.
It's important to note that electrical conductivity can be influenced by factors such as temperature and the presence of specific ions or substances in the solution. These factors may require calibration or conversion factors when measuring conductivity in specific applications.
Carbon Dioxide
CO2 is an essential component for photosynthesis, and its concentration in the atmosphere can significantly influence tomato plant growth and yield. Elevated CO2 levels can stimulate photosynthesis in tomato plants. With higher atmospheric CO2 concentrations, the rate of CO2 uptake by the plant increases, leading to enhanced carbon fixation and higher production of carbohydrates. This increased photosynthetic activity provides more energy for growth and development, resulting in improved plant biomass and overall productivity.
Tomato plants grown under elevated CO2 levels often exhibit improved water use efficiency. As CO2 concentration rises, the stomatal openings on the leaves can partially close, reducing water loss through transpiration. This helps conserve water and allows the plant to maintain better hydration, even under water-limited conditions. The improved water use efficiency can be particularly beneficial in arid or drought-prone environments.
Increased carbon availability from elevated CO2 levels supports greater leaf expansion, stem elongation, and overall biomass accumulation. This results in larger and more vigorous plants with increased leaf area, which can positively impact fruit yield. Elevated CO2 levels can advance the flowering and fruiting process in tomatoes. The enhanced carbon availability stimulates reproductive growth, leading to earlier and more synchronized flowering. This can result in earlier fruit sets and extended fruiting periods, potentially increasing the total yield.
Elevated CO2 concentrations generally have positive effects on fruit quality in tomatoes. The increased carbon availability can enhance the synthesis and accumulation of sugars, organic acids, and other flavor compounds, leading to improved taste and sensory attributes of the fruits. However, it is important to note that the effects of CO2 on fruit quality can vary depending on other environmental factors and the specific tomato variety.
The effects of elevated CO2 on tomato growth and yield can be influenced by other environmental factors, such as temperature, light intensity, and nutrient availability. The response to elevated CO2 may differ under different growing conditions and can be modified by the presence of stressors or limitations associated with other factors.
It is worth mentioning that while elevated CO2 levels can have positive effects on tomato growth and yield, these effects can vary depending on the specific concentration of CO2, duration of exposure, and other environmental factors. Additionally, the long-term implications of elevated CO2 on tomato plants and their interactions with changing climatic conditions require further research and investigation.
References
Bergougnoux, V. (2014). The history of tomato: from domestication to biopharming. Biotechnology advances, 32(1), 170-189.
Brewer, M. T., J.B. Moyseenko, A.J. Monforte, and E. van der Knaap. 2007. Morphological variation in tomato: A comprehensive study of quantitative trait loci controlling fruit shape and development. Journal of Experimental Botany, 58(6), 1339–1349. https://doi.org/10.1093/jxb/erl30
Heuvelink, E., L.T. Li Tao, and M. Dorais. 2018. ‘Crop growth and yield.’, Crop Production Science in Horticulture. CABI International. doi: 10.1079/9781780641935.0089
Kandel, D. R., Marconi, T. G., Badillo-Vargas, I. E., Enciso, J., Zapata, S. D., Lazcano, C. A., Crosby, K., & Avila, C. A. 2020. Yield and fruit quality of high-tunnel tomato cultivars produced during the off-season in South Texas. Scientia Horticulturae, 272, 109582
Ouyang, Z., J. Tian, X. Yan, and H. Shen. 2021. Effects of different concentrations of dissolved oxygen on the growth, photosynthesis, yield and quality of greenhouse tomatoes and changes in soil microorganisms. Agricultural Water Management, 245, 106579.
Rančić, D., Quarrie, S. P., & Pećinar, I. 2010. Anatomy of tomato fruit and fruit pedicel during fruit development. Microscopy: Science, technology, applications, and education, 2, 851-861.
Smith, A. F. (2001). The tomato in America: early history, culture, and cookery. University of Illinois Press.
Virginia Department of Agriculture and Consumer Services. 2020. Virginia Agricultural Statistics 2019 Annual Bulletin. Retrieved from: https://www.nass.usda.gov/Statistics_by_State/Virginia/Publications/Annual_Statistical_Bulletin/A nnual%20Bulletin_19.pdf.
Went, F. W. 1944. Morphological Observations on the Tomato Plant. Bulletin of the Torrey Botanical Club, 71(1), 77. https://doi.org/10.2307/2481487.
Virginia Cooperative Extension materials are available for public use, reprint, or citation without further permission, provided the use includes credit to the author and to Virginia Cooperative Extension, Virginia Tech, and Virginia State University.
Virginia Cooperative Extension is a partnership of Virginia Tech, Virginia State University, the U.S. Department of Agriculture, and local governments. Its programs and employment are open to all, regardless of age, color, disability, sex (including pregnancy), gender, gender identity, gender expression, genetic information, ethnicity or national origin, political affiliation, race, religion, sexual orientation, or military status, or any other basis protected by law.
Publication Date
May 31, 2023