Denitrification Management
ID
BSE-54P (BSE-347P)
What Is Denitrification?
The only permanent removal of nitrogen (N) within both soils and aquatic environments is microbial denitrification*, which reduces reactive nitrogen in the form of nitrate (NO3-) to inert dinitrogen gas (N2), which comprises approximately 78 percent of our atmosphere, or 4,000 trillion tons. Denitrification currently returns about half of excess (i.e., above plant requirements and potentially mobile) agricultural nitrogen sources to the atmosphere and could perhaps do more if effectively utilized within pollution-reduction strategies.
Soil microorganisms need oxygen to survive, and when the soil becomes saturated, oxygen is depleted, often referred to as anaerobic. Some heterotrophic microbes can get the oxygen needed to survive from nitrite (NO2-) and NO3-. When microbes utilize this oxygen, N2 gas is created in a process called denitrification. Under certain conditions partial denitrification can occur, which results in the production of nitrous oxide (N2O) or nitric oxide (NO), both powerful greenhouse gases. These gases are then lost to the atmosphere. In order to more fully understand what denitrification is and how it occurs, a brief explanation of the nitrogen cycle is warranted.
* Terms defined in the glossary at the end of this publication are italicized the first time they appear in the text.
The Nitrogen Cycle
Nitrogen is a naturally occurring element that is required by both plants and animals for growth and reproduction. As shown in figure 1, the nitrogen cycle can quickly become complex, with many nitrogen species interacting and constant transformations occurring under various conditions. The most abundant species of nitrogen — free, unreactive nitrogen in the atmosphere, dinitrogen gas — is not in a form that is readily usable by plants or animals and does not combine readily with other elements because it is very stable. Thus, a substantial input of energy is required to break the N2 bonds and create bioavailable nitrogen via nitrogen fixation. The nitrogen cycle consists of six well-understood steps and one that has been newly discovered that are discussed in greater detail below.
1. Nitrogen Fixation
Three processes are responsible for nitrogen fixation, which is the conversion of N2 into a form that can be used by plants and animals (i.e., reactive or bioavailable nitrogen).
• Atmospheric fixation by lightning. Atmospheric fixation occurs when the extreme temperature of lightning splits the nitrogen gas bond and combines with oxygen and water vapor in the air to form nitrate.
• Biological fixation by certain microbes (Rhizobia and Bradyrhizobia bacteria in root nodules), alone or in a symbiotic relationship with some plants such as legumes (e.g., soybeans, beans, peas). All biological nitrogen fixation requires the enzyme nitrogenase.
• Industrial fixation via the Haber-Bosch process used to produce fertilizer products such as urea that require a large amount of energy, primarily from non-renewable fossil fuels.
2. Immobilization
Abiotic reactions that sequester mineral nitrogen (e.g., ammonium fixation (sorption) by clay minerals) and biotic uptake and conversion of nitrogen to proteins and cell tissue by plants and microbes. Organisms mainly use nitrogen in its mineral forms (inorganic) NO3- and ammonium (NH4+), but it may also include uptake of soluble organic nitrogen. Biotic immobilization
is also referred to as accumulation.
3. Mineralization
Microbial decomposition of organic nitrogen to mineral forms (reverse of immobilization). Ammonification is a subset of mineralization that results in the formation of NH4+.
4. Ammonia Volatilization
Production of ammonia gas (NH3) from NH4+ (as a dissolved ion) in soils with high concentrations of NH4+ and a pH greater than 7.5.
5. Nitrification
Stepwise microbial oxidation of NH4 to NO2- to NO3- by the autotrophic bacteria Nitrosomonas and Nitrobacter respectively. Nitrifiers under anaerobic conditions can also produce the greenhouse gases NO and N2O.
6. Denitrification
Microbially controlled reduction of nitrate to dinitrogen gas. In this reaction, organic carbon serves as an energy source, and nitrogen oxyanions (NO3-, NO2-) or oxides (NO, N2O) serve as the terminal electron acceptor in the absence of free oxygen:
NO3- ⇒ NO2- ⇒ NO ⇒ N2O ⇒ N2
During each step organic carbon is utilized and CO2 and H2O are produced. Denitrification is the link completing the nitrogen cycle, converting reactive nitrogen (Nr) back to dinitrogen.
7. Anammox
Anaerobic oxidation of ammonium utilizing carbon dioxide (bicarbonate in aqueous environment) as an electron donor and nitrite as an electron acceptor. Anammox converts 90 percent of the reactant nitrogen to N2 while converting 10 percent to NO3. This process was discovered in a denitrifying wastewater treatment reactor in 1994. However, it is unclear how prevalent this process is in the natural environment.
In summary, the main forms of nitrogen present in the environment are nitrogen gas, organic N, ammonium/ammonia, and nitrate.
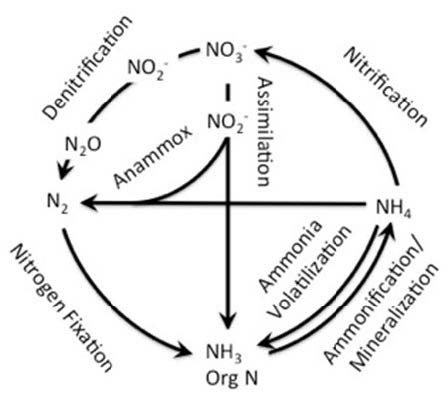
Environmental Impacts
While low N levels often limit biological production both in the terrestrial and marine environments, excess reactive nitrogen underlies a suite of environmental and health problems. Environmental degradation resulting from elevated nitrogen levels includes eutrophication of receiving water bodies, global acidification, stratospheric ozone depletion, and tropospheric accumulation of ozone and aerosols, which can lead to respiratory illness, cardiac disease, and cancer in humans.
Nitrogen can contaminate potable water. High concentrations of nitrate are toxic to infants — resulting in methemoglobinemia (blue baby syndrome) — as well as to livestock, while nitrite reacting with secondary amines forms carcinogenic nitrosamines.
Disruption of the global nitrogen cycle also has a multitude of potential impacts on the other major chemical cycles of carbon, phosphorus, and sulfur, which have become a topic of increasing study, particularly with respect to global climate change.
Thus, mitigating the effects of anthropogenic nitrogen inputs is vital to protection of our health and natural resources while continuing to produce food and energy for a growing population. Agricultural productivity is dependent on inputs of manufactured nitrogen fertilizer while energy production from fossil fuels also releases nitrogen that would not naturally be made available. Promoting and managing denitrification is paramount because the human population is sustained by anthropogenic increase in reactive nitrogen.
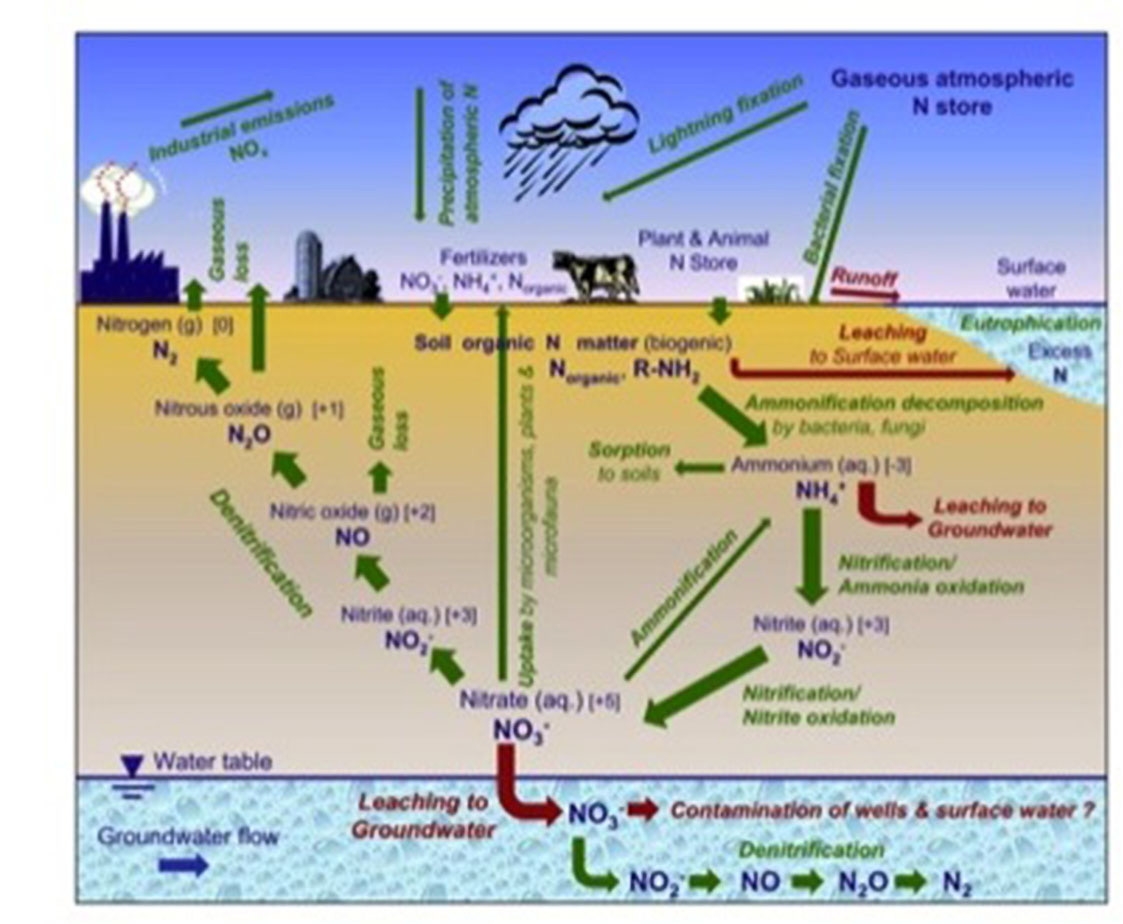
Figure 2 shows the various pathways that nitrogen can take in the environment. The fate of nitrogen depends on many conditions, including soil texture, organic matter content, soil moisture status, soil oxygen levels, soil pH, and temperature. Runoff and leaching are two, often large, pathways that transport nitrogen throughout the environment. Nitrogen runoff not only removes valuable plant nitrogen, but can also contribute to eutrophication of water bodies. Excessive nitrogen leaching also removes valuable plant nitrogen, can contaminate groundwater reservoirs and wells, and is very difficult to remove.
Incomplete denitrification or the production of harmful greenhouse gases N2O and NO can degrade air quality. Nitrogen can be taken up by plants and microorganisms, stored in the soil (as organic nitrogen), or immobilized by sorption to soil.
Denitrification Management
Denitrification management (e.g., promoting conversion of excess NO3- to N2) is thought to have some of the highest potential in agro-ecosystems. Globally, 75 percent of anthropogenic Nr is utilized in agro-ecosystems, of which approximately half is lost to the atmosphere (NH3, NO, N2O, N2) or to water (NO3-); complete denitrification to N2 accounts for only a minor portion.
Reduction of excess reactive nitrogen from a watershed scale to global scale must rely on multiple strategies employed in concert.
There are four major management categories:
1. Minimize NO and N2O emissions from fossil fuel combustion.
2. Improve nitrogen uptake efficiency in crops.
3. Increase nutrient management of livestock operations.
4. Expand access to sewage treatment globally.
Yet even these load reductions will not reduce reactive nitrogen inputs sufficiently to restore the balance in the global nitrogen cycle. The quantity of excess Nr, as well as its persistence in the environment determines its impact because as nitrogen is transported through the environment and undergoes transformation, a single molecule may have multiple negative impacts, such as eutrophication (NO3), methemoglobinemia (NO2), or as a greenhouse gas (N2O, NO). This concept, known as the “nitrogen cascade,” emphasizes the importance of balancing anthropogenic nitrogen inputs with denitrification. Denitrification management — promoting reactive nitrogen removal throughout the cascade — is increasingly recognized as an essential component of managing the global nitrogen cycle, especially with respect to diffuse pollution.
Restoration and construction of wetlands and riparian buffers for nitrogen removal are examples of denitrification management applicable to a wide range of landscapes. However, many areas of our landscape are natural denitrification hot spots.
Denitrification occurs primarily under anaerobic conditions by heterotrophic microbes and, assuming sufficient NO3-, denitrification rates are high in wet, carbon-rich soils.
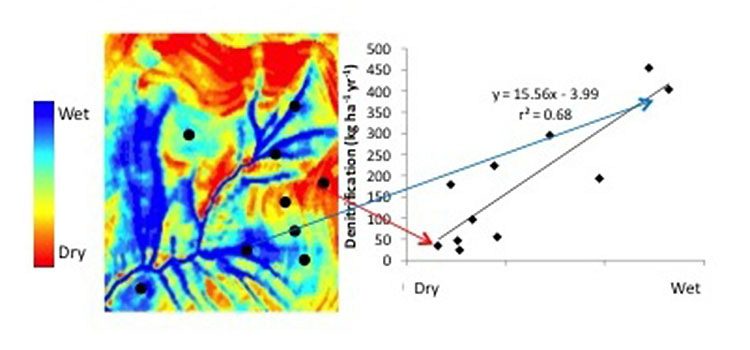
Figure 3 shows results from a field study of denitrification potential across a landscape. Notice in this figure that the highest denitrification rates are found in areas of the landscape that are most likely to be saturated (i.e., wet, often anaerobic or oxygen deficient). These denitrification hot spots also tend to be riparian areas with minimal disturbance, where soil-organic carbon can accumulate (remember, carbon is also a critical component in because it serves as the energy source to drive denitrification).
Limitations and Unknowns
Partial Denitrification
Under certain circumstances, harmful greenhouse gasses such as NO and N2O can be produced, and NO2- reacts to form a carcinogen.
Significant greenhouse gas production has been observed in other nitrogen removal systems utilizing denitrification, including constructed wetlands, which have been shown to act as both sources and sinks of nitrogen gases, occasionally producing high emissions of N2O.
Increased temperature and certain carbon types — such as those with more easily utilized carbon content — can increase N2O production during denitrification.
What You Can Do
• Protect areas of the landscape that act as natural denitrification hotspots. These would include
Figure 3. Data from denitrification field study. The left figure indicates areas of the landscape likely to be saturated (i.e., anaerobic), with black dots representing sampling locations. The plot on the left is of denitrification rates from those sampling locations. Notice that the denitrification rates are substantially higher in the wet areas. frequently saturated areas, riparian areas, and wetlands.
• Plant residue is an important source of carbon and nitrogen for nitrification and denitrification. Increasing soil carbon content has the potential to provide in-field denitrification under certain conditions.
• Increase water residence time and contact with biological surfaces in ditch networks. Allowing some vegetation to grow, which slows water velocity and provides carbon, can do this.
• Fertilizer type can influence the magnitude of nitrification and denitrification and, as a result, nitrogen fluxes. For example, injected anhydrous ammonia commonly has higher NO production than other fertilizers.
• Recent work suggests that the use of controlled drainage in fields with subsurface tile drains can both prevent offsite transport of excess nitrogen and provide increased denitrification during periods of prolonged saturation.
Acknowledgements
We would like to thank David Sample, professor and Virginia Cooperative Extension specialist, Virginia Tech; Joshua Faulkner, assistant professor and West Virginia University Extension specialist; and David Moore, Virginia Cooperative Extension agent and unit coordinator, Middlesex County, for their helpful and insightful reviews.
Additional Resources
Food and Agriculture Organization of the United Nations (FAO), Natural Resources Management and Environment Department, and the International Fertilizer Industry Association. 2001. “Factors Regulating Nitrous Oxide and Nitric Oxide Emission.” Chapter 2 in Global Estimates of Gaseous Emissions of NH3, NO and N2) From Agricultural Land. Rome: FAO. www.fao.org/docrep/004/y2780e/y2780e02.htm.
National Academy of Engineering Grand Challenges for Engineering – www.engineeringchallenges.org/cms/8996/9132.aspx
References
Rivett, M. O., S. R. Buss, P. Morgan, J. W. N. Smith, and C. D. Bemment. 2008. “Nitrate Attenuation in Groundwater: A Review of Biogeochemical Controlling Processes.” Water Research, 42(16): 4215-32.
Glossary of Terms
Abiotic – Nonliving factors that affect living organisms.
Anaerobic – Chemical reactions that proceed without the presence of oxygen.
Biotic – Consisting of living organisms.
Bradyrhizobia – See Rhizobia.
Denitrification – The transformation of nitrates to nitrites, nitric oxide, nitrous oxide, and ultimately, dinitrogen gas, which is lost to the atmosphere (i.e., the nitrogen cycle). Denitrification is fostered by anaerobic (see Anaerobic), carbon-rich conditions.
Eutrophication – Water body response to excess nutrients like nitrogen and phosphorus, generally resulting in excessive plant growth that often results in hypoxia — the depletion of oxygen in the water.
Global acidification – Also referred to as ocean acidification, it is the process of the current and ongoing decrease in the pH of the ocean. While increasing atmospheric carbon dioxide (CO2) levels are the primary cause of ocean acidification, nitrogen oxides (N2O, NO) also contribute substantially. The pH is reduced when these oxides are dissolved in seawater, which causes an increase in the hydrogen ion (H+) in solution (note that H+ is acidic and lowers the pH):
(CO2, NO, N2O) (aq) + H2O <->H2CO3 <->HCO3- + H+ <->CO32- + 2 H+
Greenhouse gases – A compound in the earth’s atmosphere that blocks or traps heat that would normally radiate out of the atmosphere. The primary greenhouse gases are carbon dioxide, methane, nitrous oxide, and ozone.
Heterotrophic microbes – Microbes that obtain energy, carbon, and reducing equivalents for reactions from organic compounds.
Labile – The ability of a substance change easily. Labile carbon would mean carbon that is easily digested or utilized by microbes.
Leaching – The movement of water or dissolved constituents, usually downward; also referred to as percolation, out of the root zone.
Methemoglobinemia – Acute poisoning, primarily of infants, caused by nitrate ingested at high concentrations. Nitrate is converted to nitrite in an infant’s digestive system, and the nitrite reacts with hemoglobin (that carries oxygen to all parts of the body) to form methemoglobin, which does not carry oxygen. The level of oxygen being carried throughout the body decreases as hemoglobin is converted to methemoglobin. As the oxygen level decreases, the baby is suffocated.
Microbial decomposition – The breakdown of compounds or organic matter into smaller compounds by microorganisms.
Nitrobacter – A bacteria that converts nitrite into nitrate.
Nitrogenase – Enzymes used to fix atmospheric nitrogen gas (N2) to convert to ammonia (NH3).
Nitrosomonas – A bacteria that converts ammonia into nitrite.
Rhizobia – Soil microbes that fix nitrogen once established in legumes. The microbes maintain a symbiotic (i.e., both microbe and plant benefit) relationship with the legume. The microbes supply ammonia or amino acids to the plant and, in return, receive organic acids as a carbon and energy source.
Riparian – Describes the near stream area, often forested, and subject to periodic saturation.
Sorption – The physical bonding of one substance to another.
Virginia Cooperative Extension materials are available for public use, reprint, or citation without further permission, provided the use includes credit to the author and to Virginia Cooperative Extension, Virginia Tech, and Virginia State University.
Virginia Cooperative Extension is a partnership of Virginia Tech, Virginia State University, the U.S. Department of Agriculture, and local governments. Its programs and employment are open to all, regardless of age, color, disability, sex (including pregnancy), gender, gender identity, gender expression, genetic information, ethnicity or national origin, political affiliation, race, religion, sexual orientation, or military status, or any other basis protected by law.
Publication Date
February 28, 2023