Irrigation Considerations for Commercial Hop Producers
ID
SPES-95 (SPES-622NP)
EXPERT REVIEWED
Introduction
Supplemental irrigation is the delivery of additional water above what is received through normal precipitation to support plant growth and production. Hops are a high-value crop, and both yield and quality are affected when they are subjected to water stress. In many climates, including the Mid-Atlantic region of the U.S., supplemental irrigation is required for economical production of hops.
Many factors affect the amount and frequency of supplemental irrigation required, including climate (precipitation, temperature, relative humidity, etc.), soil characteristics (soil type, slope, depth, water-holding capacity, etc.), and plant-specific characteristics (including stage of development and plant biomass).
This publication provides an overview of the many factors that influence irrigation management decisions for hops, as well as an overview of the steps involved in developing an irrigation program for individual hop yards.
Hop Growth and Development Characteristics Influencing Water Use
Hops are perennial plants usually grown on a tall trellis system 15-20 feet high. Each spring, new shoots emerge from the underground crown, with 2-3 shoots (bines) being trained to coir strings in mid-to-late spring (figure 1). The trained bines continue elongating and climb vertically until days begin to shorten following the summer solstice (around June 21). During this period of rapid vertical growth, side shoots develop and plant biomass increases significantly. Following the summer solstice, plant energy shifts from vegetative growth to reproduction, and flowers begin to develop. These early flowers (burrs) progress into cones, which continue growing until they reach maturity in late July to early September. Cones are mature when they reach a dry matter content of 22-25 percent, at which point the bines are harvested.
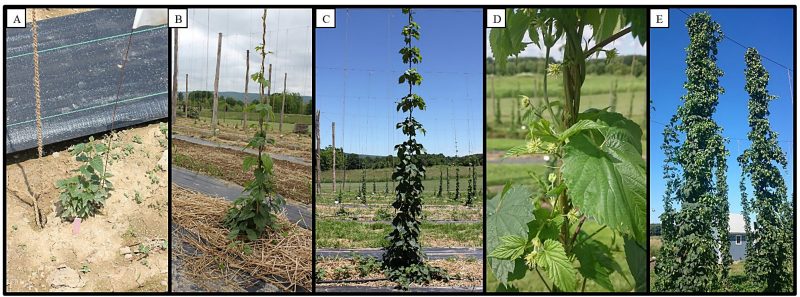
One of the major factors influencing water requirements is the rate of transpiration — the loss of water through the leaf stomata. As expected, this rate is highly correlated with plant biomass. As a plant grows and biomass increases through the season, so does a plant’s rate of transpiration and its water requirement. Due to the growth cycle of hops and the large seasonal variability in biomass, the water requirement varies significantly depending on the development stage. Previous reports estimate 75-80 percent of the water that hops require each season is needed between July and August, when plants are at peak biomass (Evans 2003; figure 2).
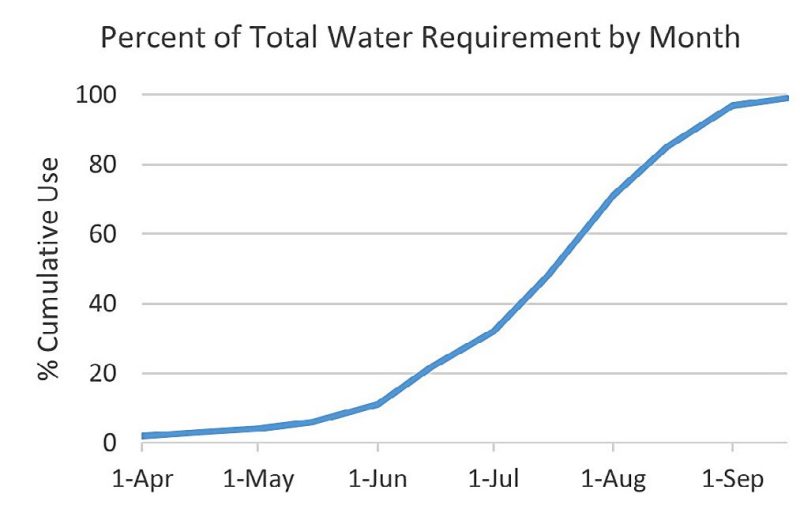
Climate
Many climatic factors influence a crop’s water use requirement, but the most important is the amount, frequency, and timing of rainfall. Previous reports suggest most hop cultivars in the Pacific Northwest require 24-28 inches of water per growing season (Evans 2003). In much of the state of Virginia, average rainfall commonly exceeds 18-20 inches during the five- month (April-August) growing season for hops (Table 1). Although hops grown in Virginia receive the majority of their required water through normal seasonal rainfall, periods of a week or more without precipitation are common, which can stress plants and impact production. Additionally, summer rains can be heavy and exceed soil water-holding capacity, causing a portion of the precipitation received to be leached through the soil or lost as runoff.
Additional climatic considerations, including temperature, relative humidity, and wind, all influence plant water requirements, as they all affect the rate of transpiration. These same climate characteristics also influence the loss of moisture from the soil by evaporation, which, collectively with the rate of transpiration, makes up the evapotranspiration rate.
Impacts of Soil Characteristics on Irrigation
The physical and chemical properties of the soil play a significant role in a crop’s irrigation requirement by influencing the nutrient- and water-holding capacities of a soil. The primary factor influencing water-holding capacity is soil texture, which is determined by the composition of particles within the soil less than 2 millimeters in size. These particles include sand (greater than 0.05 mm), silt (0.05 – 0.002 mm) and clay (less than 0.002 mm). The ratios of these particles to one another within a soil are used to characterize the soil based on the dominant particle types (figure 3).
Location |
April |
May |
June |
July |
August |
Season Totala |
Annual |
---|---|---|---|---|---|---|---|
Blacksburg1 |
3.81 |
4.09 |
3.66 |
4.01 |
3.47 |
19.04 |
40.61 |
Charlottesville2 |
3.43 |
4.19 |
4.40 |
4.96 |
4.55 |
21.53 |
45.97 |
Danville3 |
3.45 |
3.89 |
3.73 |
4.42 |
3.96 |
19.45 |
42.81 |
Lynchburg4 |
3.12 |
3.57 |
3.76 |
4.19 |
3.73 |
18.37 |
40.95 |
Norfolk5 |
3.12 |
3.62 |
3.88 |
5.37 |
5.48 |
21.47 |
45.68 |
Richmond6 |
3.02 |
3.70 |
3.70 |
4.86 |
4.85 |
20.13 |
43.64 |
Washington, DC6 |
2.95 |
3.77 |
3.51 |
3.82 |
3.86 |
17.91 |
39.68 |
Winchester7 |
3.12 |
3.76 |
3.90 |
3.76 |
3.64 |
18.18 |
37.63 |
Source: The Southeast Regional Climate Center, University of North Carolina at Chapel Hill, http://www.sercc.com/
Data represents the period between: 1(1952-2012), 2(1893-2012), 3(1891-2012), 4(1930-2012), 5(1946-2012), 6(1948-2012), and 7(1912-2012).
a Seasonal totals are the sums of average monthly precipitation during the five-month growing season for hops (April-August).
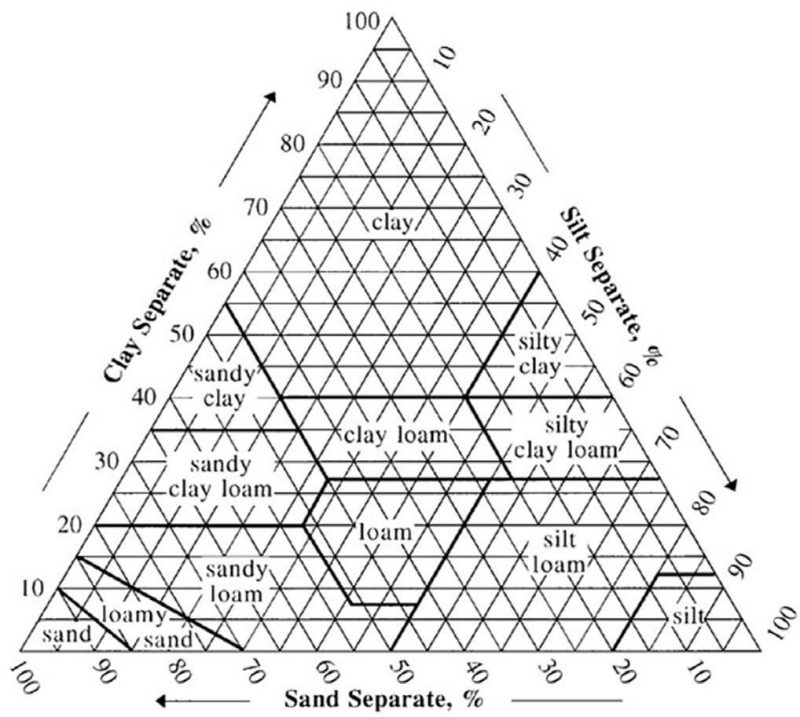
When soils are saturated following irrigation or rain, the space between soil particles is filled with water, displacing air and oxygen (figure 4). Under these conditions, gravity is the primary force on the water as it moves downward through the soil pores. After the soil has drained for a period, it will reach what is known as field capacity, which is the maximum amount of water a soil can hold due to capillary forces. At field capacity, capillary forces (cohesion and adhesion) hold water between adjacent soil particles, and air begins to fill any voids between particles. Under these conditions, water is readily accessible to the plant roots, and the amount of plant energy exerted to overcome soil capillary force is minimal.
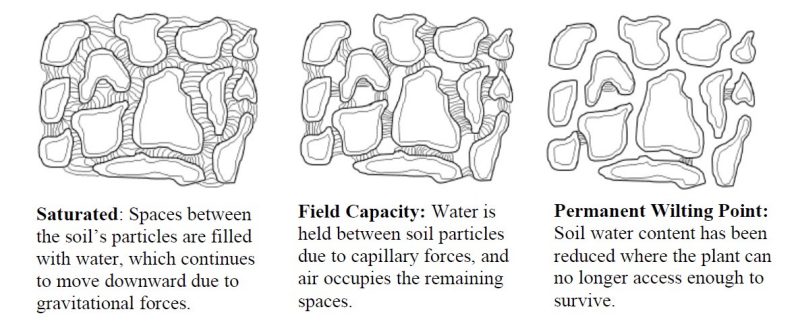
However, if the soil continues to dry due to evaporation or removal by the plant, the soil moisture level will reach the permanent wilting point. When the permanent wilting point is reached, the energy required to remove the remaining water molecules within the soil is greater than the energy the plant can exert. The water contained in the soil above field capacity quickly moves through the soil due to gravity and is inaccessible to the plant roots. Likewise, the energy required to access the moisture remaining after the permanent wilting point is reached is more than the plant can expend. Therefore, the volume of water the soil can hold between field capacity and the permanent wilting point is known as the plant-available water (figure 5).

The size of the specific particles within the soil determines the amount of space between particles, known as the porosity. Soil texture greatly influences the volume of water the soil contains per unit volume at field capacity, which is also known as water-holding capacity. Soils that contain larger particles, like sand, have more space between particles, while much smaller clay particles fit closely together and have little space between them. As a result, sand particles have less surface area (per unit volume), exerting less capillary forces on water molecules than smaller clay soil particles; therefore, soils containing high sand content
have lower water-holding capacity. Stated another way, clay particles are smaller and therefore the space between particles is also smaller and water molecules are held more tightly in these small spaces, resulting in clay soil having a much higher water-holding capacity. For an in-depth discussion, see Soil and Soil Water Relationships, Virginia Cooperative Extension publication BSE-194P, available online at https://pubs. ext.vt.edu/BSE/BSE-194/BSE-194.html (Easton and Bock 2016).
Another import characteristic to consider when establishing a hop yard or irrigation program is soil depth. Shallow soils are those that contain confining layers — layers of materials that block or slow the flow of water — near the rooting zone. These shallow soils have a reduced water-holding capacity and dry out quicker; therefore, they require more frequent irrigation in order to avoid plant stress. This is particularly important to consider for deeply rooted plants like hops.
Additional Irrigation Considerations
Managing soil moisture is a delicate balancing act. When a crop’s need for water is greater than the water available, it is unable to execute vital functions and plant growth is suspended. This results in plant stress and may lead to lower yields and even plant death. Symptoms of water stress include decreased plant vigor, suspended growth, drying or brittle leaves, or in extreme cases, plant wilting.
Conversely, too much water can also be problematic. When the soil is excessively wet due to over-irrigation, prolonged precipitation, or poor drainage, nutrients may leach through the soil, resulting in negative economic impacts as well as environmental damage due to nutrient contamination of groundwater. Additionally, prolonged periods of soil saturation and anoxic conditions within the plant rooting zone promotes root rot and fungal disease.
When plant water demand and available soil moisture are equal, plant stress is avoided and soil nutrients remain within the rooting zone.
Types of Irrigation Systems
The three primary irrigation system designs used in large field crop plantings are overhead, furrow, and drip.
Overhead irrigation systems, such as broadcast or sprinklers, disperse water above the plant canopy, allowing water droplets to fall through the plant canopy and continue to the soil surface. Although commonly used for many field crops, due to the spacing of hop plants, overhead irrigation systems are generally avoided because they have very low water-use efficiency.
Additionally, overhead irrigation systems are also not advisable for hop producers due to the increased risk of disease that results from prolonged leaf wetness.
Furrow irrigation systems use a channel or furrow between rows that can be flooded, allowing water to infiltrate through the soil into the plant rooting zone. These systems have a lower water-use efficiency because of surface runoff and deep percolation through the soil. Due to their design, furrow irrigation systems produce large swings in soil moisture as the soil fluctuates between saturation and depletion, and so they may result in plant stress.
Drip irrigation systems supply water through lines running the length of plant beds. They may contain emitters, or intermittently spaced openings, along the irrigation line, and they apply water directly to the soil adjacent to plants (figure 6). These systems have a much higher water-use efficiency than either furrow or overhead irrigation systems, allowing the grower to maintain precise control over soil moisture. Drip systems also allow for the delivery of fertilizer solutions directly to plant root zones.
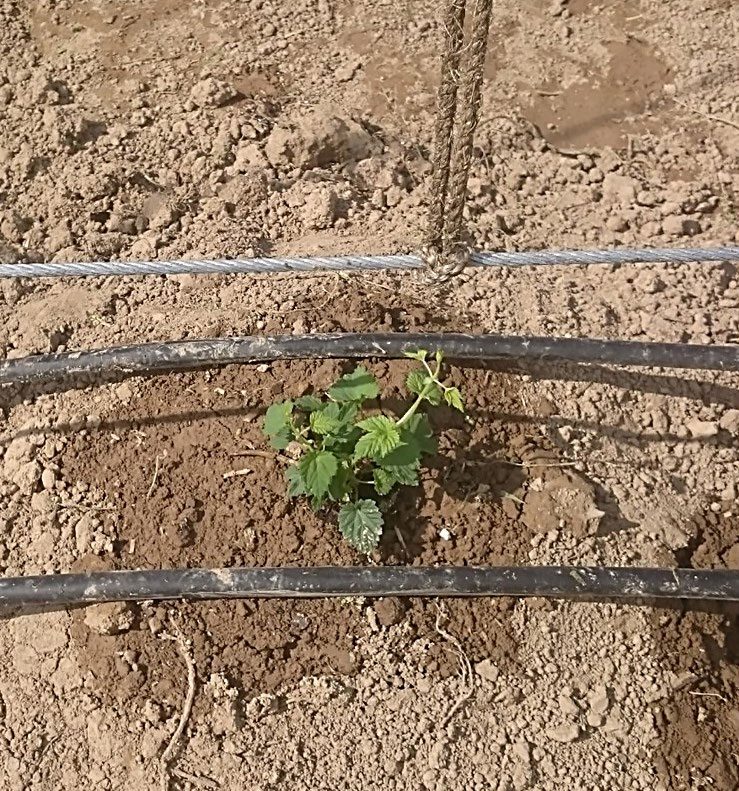
Sources of Irrigation Water
The primary sources for irrigation water are surface water, including streams, lakes, and ponds, and groundwater, in which irrigation wells are drilled to access aquifers or saturated underground layers. Many factors should be considered before installing an irrigation system, including crop demand, discharge rate, and seasonal water availability. For example, if an irrigation well’s discharge rate is less than a crop’s demand, plants will be stressed, and production could suffer. Additionally, peak water demand for hops occurs during the late summer (July-August) when streams and ponds are generally at their lowest, potentially making water availability an issue. Alternative water sources should be considered, or arrangements should be made for storage tanks or other ways to increase an operation’s water-holding capacity.
Water Quality
Before establishing an irrigation system, it is important to understand local water characteristics, which may vary widely between regions due to differences in geology, land use, and climate. Although many potential contaminants could impact the quality of irrigation water and thus the productivity/safety of the crop, the most common issues are related to pH, alkalinity, and electrical conductivity (dissolved salts) in the water.
Maintaining good water quality is especially important for drip irrigation systems. For more information on water quality in drip irrigation systems, please see Virginia Cooperative Extension Publication 442-757, “Filtration, Treatment, and Maintenance Considerations for Micro-Irrigation Systems.”
Chemically, pH is the concentration of hydrogen ions (H+) in solution and is measured on a scale of 0-14. Solutions with high concentrations of hydroxides have a pH below 7 and are acidic, while solutions with low concentrations of hydroxides are basic and have a pH above seven. Plants prefer solutions with relatively neutral to slightly acidic pH (5.6-6.2), as significant variations outside this range in either direction may result in nutrient issues (toxicity or deficiencies).
Alkalinity is the dissolved carbonates and bicarbonates in water and influences a solution’s ability to absorb acids. It is also known as “buffering capacity.” Solutions with high alkalinity and high pH will resist corrective actions taken to lower pH (adding acids).
Additionally, irrigating using water sources with high levels of carbonates may lead to some of these compounds settling out in the irrigation system, which could clog emitters.
Electrical conductivity, abbreviated as EC, is the measure of the dissolved salts (ions) in water. Solutions high in salts can impair the plant roots’ ability to take up water, which negatively affects plant growth. High EC in water can also indicate contamination from salts, such as fertilizers or other sources, and should be monitored. If well water is used in coastal areas, the EC in particular should be monitored regularly due to the potential for saltwater intrusion into groundwater. For more information about regional groundwater characteristics in Virginia, see Using Groundwater for Agricultural Irrigation in Virginia, VCE publication BSE-215P, available online at https://pubs.ext.vt.edu/ BSE/BSE-215P/BSE-215P.html (Shortridge 2017).
Monitoring Soil Moisture
Many types of soil moisture meters are available to help growers maintain proper soil moisture. Most of these sensors operate using two basic functions: measuring soil tension (suction) or determining soil water volume by electrical signal transmission. Figure 7 shows several types of soil moisture sensors. The following sections provide a brief overview on methods for monitoring soil moisture. For additional details on soil moisture sensors, please see Virginia Cooperative Extension Publication BSE-338P, “Soil Moisture Sensors for Agricultural Irrigation: An Overview on Sensor Type.” For additional information about using the data generated by sensors for irrigation management, please see BSE-339P, “Scheduling Agricultural Irrigation Based on Soil Moisture Content: Interpreting and Using Sensor Data.”
The most common soil tension meters are tensiometers. These sensors are composed of a tube filled with water, with a porous cup on one end that is saturated and installed into the soil. As soil moisture is depleted, the water within the sensors is pulled through the porous cup, creating negative pressure on the tube that is signaled on a pressure gauge. As the soil moisture continues to decrease, the vacuum (negative pressure) on the solution within the sensor increases. Tensiometers can be incorporated into an automated irrigation system, which is activated by a magnetic switch when the gauge reaches a predetermined set point.
Many types of sensors measure soil water volume. In general, these sensors work using a probe or multiple probes inserted in the soil that emit a signal (usually electrical current) and measure the strength or duration between signal responses, which is proportional to the water content in the soil over a specific distance. For more information on soil moisture sensors, see Understanding Soil Moisture Sensors: A Fact Sheet for Irrigation Professionals in Virginia, VCE publication BSE-198P, available online at https://pubs.ext.vt.edu/ BSE/BSE-198/BSE-198.html (Sample et al. 2016).

Calculation of Hop Irrigation Requirement
Evans (2003) reports most hop cultivars grown in Washington State require 24-28 inches of water per season. If this rate were divided evenly over the five- month growing season, it would equate to about 1.4 inches of water per week. However, due to the growth cycle of hops, the majority of the crop water demand occurs in late summer (figure 2). Other reports suggest hops require 1 to 1.4 inches of water applied twice per week during hot and dry weather (Elford 2011). Using 2 inches total per week as a target, it is possible to begin calculating the irrigation system requirements of a hop yard.
In the Virginia Tech hop yard, hops are planted every 3.5 feet within rows, with 12 feet between rows. Hop roots are pruned periodically from between the rows to avoid crown creeping. Consequently, each plant occupies roughly 12.25 square feet (3.5 feet by 3.5 feet) within the hop yard.
To calculate how much irrigation is needed to provide 2 inches of water across a large area, it’s helpful to know these statistics:
Acre-inch = 27,158 gallons (amount of water required to cover one acre of land in one inch of water)
Area in one acre = 43,560 square feet
Therefore, the volume of water required to provide 1 inch of water per square foot = 0.62 gallons (27,158 gallons divided by 43,560 square feet).
If hop plants take up 12.25 square feet of land, they require up to 15.2 gallons of water per plant per week:
12.25 square feet per plant x 2 inches per week x 0.62 gallons per square foot = 15.2 gallons.
The Virginia Tech hop yard uses a drip irrigation system with emitters every 12 inches within the row, each discharging 1.15 gallons of water per hour of operation. With hop plants spaced every 3.5 feet within the row, each plant has access to at least three emitters. Thus:
15.2 gallons per week per plant ÷ 3 emitters per plant ÷ 1.15 gallons per emitter per hour = 4.4 hours of irrigation per week
Therefore, if no rainfall occurs, the hops should be irrigated for a minimum of 4.4 hours per week.
However, irrigation events should be scheduled based on soil moisture readings, because if the entire 15.2 gallons of water were supplied at once, the soil’s water-holding capacity would be exceeded, resulting in a portion of the water being lost to either leaching or runoff. This wastes irrigation water and potentially leads to water stress if that portion of water is not replaced later in the week. This may mean in areas with sandier soils and low field capacity, irrigation events should occur more frequently with less volume delivered at a time. In areas with heavier, more clay or loamy soils, the soil’s water- holding capacity is much higher and can accept large volumes of water at each irrigation event.
Hop plants are a high-value crop that benefit from irrigation, especially during the hottest weeks of the growing season. Hop growers who decide to irrigate need to consider their farm’s soil type, different irrigation systems, and various soil moisture monitors, and then take steps to make sure they don’t over- or under-irrigate.
References
Easton, Z. and E. Bock. 2016. “Soil and Soil Water Relationships.” VCE publication BSE-194P. https:// pubs.ext.vt.edu/BSE/BSE-194/BSE-194.html.
Elford, E. 2011. “Irrigation Requirements for your Hop Yard.” Ontario Ministry of Agricultural, Food, and Rural Affairs, OnSpecialtyCrops blog. https://onspecialtycrops.wordpress.com/2011/07/21/ irrigation-requirements-for-your-hop-yard/
Evans, R. 2003. “Hop Management in Water-Short Periods.” Washington State University Extension. Fact Sheet EM4816. http://cru.cahe.wsu.edu/CEPublications/em4816/em4816.pdf.
Kramer, P. J., and J. S. Boyer. 1995. Water Relations of Plants and Soils. San Diego: Academic Press.
Sample, D., J. Owen, J. Fields, and S. Barlow. 2016. “Understanding Soil Moisture Sensors: A Fact Sheet for Irrigation Professionals in Virginia.” VCE publication BSE-198P. https://pubs.ext.vt.edu/ BSE/BSE-198/BSE-198.html.
Shortridge, J. 2017. “Using Groundwater for Agricultural Irrigation in Virginia.” VCE publication BSE-215P. https://pubs.ext.vt.edu/BSE/BSE-215P/ BSE-215P.html.
Shortridge, J. and B. Benham. 2018. “Filtration, Treatment, and Maintenance Considerations for Micro-Irrigation Systems.” VCE Publication 442- 757.
Shortridge, J. and W. Porter. 2021. “Scheduling Agricultural Irrigation Based on Soil Moisture Content: Interpreting and Using Sensor Data.” VCE Publication BSE-339P. https://www.pubs.ext.vt.edu/BSE/BSE-339/.
Shortridge, J. and W. Porter. 2021. “Soil Moisture Sensors for Agricultural Irrigation: An Overview on Sensor Types.” VCE Publication BSE-338P. https://www.pubs.ext.vt.edu/BSE/BSE-338/BSE-338.html.
USDA-NRCS (U.S. Department of Agriculture-Natural Resources and Conservation Service). n.d. The Southeast Regional Climate Center, University of North Carolina at Chapel Hill. http://www.sercc.com/. Accessed May 20, 2018.
USDA-NRCS (U.S. Department of Agriculture-Natural Resources and Conservation Service). https://www.nrcs.usda.gov/wps/portal/nrcs/detail/soils/edu/?cid=nrcs142p2_054311. Accessed February 20, 2019.
Acknowledgments
Thanks to Brad Bergefurd (Ohio State University Extension), Kathleen Reed and Jon Vest (ANR Agent Faculty, VCE), and John Freeborn (School of Plant and Environmental Sciences, Virginia Tech), for their comprehensive review of this Extension publication. Support for our work is provided, in part, by Virginia Agricultural Council, Virginia Department of Agriculture and Consumer Services, and the U.S. Department of Agriculture.
Virginia Cooperative Extension materials are available for public use, reprint, or citation without further permission, provided the use includes credit to the author and to Virginia Cooperative Extension, Virginia Tech, and Virginia State University.
Virginia Cooperative Extension is a partnership of Virginia Tech, Virginia State University, the U.S. Department of Agriculture, and local governments. Its programs and employment are open to all, regardless of age, color, disability, sex (including pregnancy), gender, gender identity, gender expression, genetic information, ethnicity or national origin, political affiliation, race, religion, sexual orientation, or military status, or any other basis protected by law.